Crystal Structure, Optical and Electrical Characteristics of Rutile
Crystal structure, optical and electrical characteristics of rutile
TiO2- based photoanodes doped with GeO2
The effect of germanium dioxide GeO2 doping on dye-sensitized solar cells (DSSCs) TiO2 nanocrystallites photoanodes with different concentrations with composition
Order custom essay Crystal Structure, Optical and Electrical Characteristics of Rutile with free plagiarism report
(TiO2-(GeO2)x: 0 * x 0.3 wt%)
has been studied. The samples have been prepared by a solid-state reaction method and examined by means of the X-ray diffraction (XRD) and UV-visible spectroscopy techniques. The photovoltaic characteristics for the prepared samples have been studied by employing J–V measurements. XRD spectrum depicted that the main phase in the sample with x = 0 is a tetragonal one of the rutile - type with P42/mnm space group and increasing in GeO2 ratio leads to appearance and rise of another orthorhombic phase Ge4Ti5 with Pnma space group.
Data obtained from the UV-visible spectroscopy measurements reflect that the optical energy gap (Optical) increases with increasing GeO2 content while the optical refractive index decreases. J–V photovoltaic characteristics confirms that the DSSCs co-doped with low concentration doping x = 0.05 and 0.1 of GeO2 have high power conversion efficiency, fill factor (FF), and short circuit current density (Jsc) in contrast samples with high concentration doping x = 0.2 and 0.3 of GeO2. The present results showed that the (TiO2-(GeO2)x with x =0.05 and 0.1 wt%) films are potential candidates for optical filter materials, optoelectrical, and photo-conversion energy devices.
Keywords: TiO2 nanocrystallites; rutile – type; optical energy gap; refractive index; efficiency.
Introduction
Recently, the enhancement of the efficiency of dye-sensitized solar cells (DSSCs) has been devoted by many researchers [1]. The most effectively utilized wide bandgap oxide material is still titanium dioxide TiO2 among other wideband semiconductor oxides such as ZnO, SnO2, Nb2O5, and SrTiO3, this is for its stability, non-toxicity and exceedingly refractive nature [2]. The improvement of progressed photovoltaic anode materials that successfully use solar energy from the visible region is an appealing challenge, especially for DSSC applications, as the light absorption and charge collection take place at the dye-sensitized mesoporous photoanode [3, 4].
One of the compelling methods to improve the power conversion efficiency of TiO2 DSSCs photoanodes is by doping foreign transition or alkaline earth ions into the TiO2 lattice [5–10]. The transition ions lead to expanding the absorption edge of TiO2 to the visible-light region, by embedding a new brand into the unique bandgap. On the other hand, alkaline earth ions change the crystal lattice, providing TiO2 with characteristic physical and electronic structures. Previously, TiO2 films have been doped by Ag and La. These films were sensitive to visible light and have high photocatalytic activity [11]. From the base knowledge, in the visible light region, TiO2 nanoparticles had higher catalytic activity when it doped by silver and strontium. This is due to the intensive effect caused by narrow bandgap and improvement in charge separation [12].
When TiO2 doped with chromium and antimony, absorption bands in the visible-light region of light and possessed 2.4 and 2.2 eV of energy gaps for chromium and antimony, respectively [13]. Films of TiO2 doped with Ta and Ni were synthesized; it found that these films were sensitive for the visible-light and the charges able to transfer from the electron donor levels to the conduction bands of the host materials [14]. Co and Nb co-doped TiO2 films were performed via pulsed laser deposition on (001) single crystal LaAlO3, dilute Nb doping significantly enhances the conductivity and microstructure of the TiO2 anatase film [15]. By the hydrothermal method, Zn and Mg co-doped TiO2 have been synthesized and these films claimed that the DSSCs depend on the co-doped TiO2 electrode performed high power conversion efficiency [16]. The main aim of the present work is to study the effect of germanium dioxide (GeO2) doping on the TiO2 photoanodes for DSSCs applications in order to improve the properties of TiO2 nanostructured and to enhance the photovoltaic efficiency. In addition, this study illustrates the effect of GeO2 doping in the range 0-0.3 mass fraction on the structure, optical, and electrical properties of rutile TiO2.
Experimental Technique
Titanium oxide TiO2 paste was prepared by grinding 2gm of nanocrystallites TiO2 (Aldrich 99.995%) with acetylacetone (0.25 ml) and triton X-100 (0.25ml) using a mortar for 60 minutes. Doctor - blade method was employed to coat the transparent conducting glass FTO with the paste and then annealed at 450°C for 30 min. Subsequently, the samples were soaked in an ethanol solution of N-719 dye for 24 h at room temperature and then gently splashed by ethanol. The DSSCs samples were accumulated and the electrolyte KI2 was added into the aperture by repeated addition of electrolyte drops see ref. [17]. The crystal structure and phase purity of powder samples were examined by powder X-ray diffraction at room temperature using Bruker D8 Advance diffractometer with CuK radiation = 1.54056 Å. UV–vis measurements have been carried out by using Jasco V-576 (Japan) model double-beam spectrophotometer in the range of = 190 to 1100 nm.
The J-V curves of DSSCs were carried out using the current amplifier (Keithley 427), multimeter (Aplab 1087), and data acquisition (DataQ: DI-158U). The DSSCs devices were irradiated with a homemade solar simulator with a xenon lamp (35 W), halogen lamp (55 W), and equipped with IR and UV filters to irradiate DSSCs effective area of 0.35 cm2. The power of the simulator was measured by using Lutron (SPM-1116SD) solar power meter.
Results and discussion
XRD patterns and lattice parameters
Figure 1 displays the powder X-ray diffraction patterns for the samples Tio2-(GeO2)x with 0 x 0.3 wt%. From Fig.1, one can notice that the main phase in the sample with x = 0 is a tetragonal one of the rutile - type with the P42/mnm space group. Doping of GeO2 into the material leads to the appearance and rise of an orthorhombic phase Ge4Ti5 with the Pnma space group [18] (marked with asterisks in Fig. 1. The volume fraction of the orthorhombic phase Ge4Ti5 increases with the increase of the GeO2 content. As seen from the insertions in Fig. 1, there are no shifts of peaks (101) and (110) belonging to the first rutile tetragonal phase with increasing GeO2. This indicates that there is no change in the lattice parameters a, c of rutile tetragonal phase with increasing GeO2.
Fig.2 shows that Rietveld refinement of diffraction pattern for the samples with x = 0, 0.05 and 0.3. As seen from Fig.2, the sample with x = 0 contains only the peaks of the main rutilte tetragonal phase (space group P42/mnm). In addition, with increasing GeO2 content (x = 0.05 and 0.3), the samples are observed to contain two phases; the main rutile tetragonal phase (space group P42/mnm) and another orthorhombic phase Ge4Ti5 (space group Pnma) [18]. The concentration dependences of lattice parameters a and c of rutile TiO2 tetragonal phase of TiO2-(GeO2)x samples are shown in Table 1. It is clearly seen that there is no change in the lattice parameters a and c with increasing GeO2 content. In order to show the effect of GeO2 on the crystalline structure of TiO2
Optical Measurements
The spectra distributions of absorbance for the studied films in the wavelength range 200–1100 nm are illustrated in Fig.3. UV-vis spectra depict that the basic absorption of Ti–O bond in UV light range around 400 nm, which is consistent with a bandgap of rutile TiO2 (3.0 eV) [21], while the absorption spectra of TiO2-(GeO2)x films are successfully extended to a visible wavelength range around 650 nm. The spectral distribution of transmittance T and reflectance R for the studied films in the wavelength range 200–1100 nm are depicted in Fig.4. The spectra show that high transmittance values of the films in the visible region, this reveals that the films have good homogeneity. The transmission edge appears not depending on GeO2 concentration in the films.
The presence of such an edge in the TiO2-GeO2 films suggested that it can be considered as a good optical filter material. The transmittance T and reflectance R) spectra were characterized by homogeneity and uniform thickness, this is due to the absence of ripples in the obtained spectra. In fact, the absorption coefficient spectrum near the fundamental edge is very useful to investigate the type of optical transition [22]. Therefore, the optical bandgap can be obtained from the dependence of the absorption coefficient on the photon energy (h). Band-to band transitions theory used to analyses the absorption coefficient at the fundamental edge for the organic materials [22,23].
The absorption data follow a power-law behavior which is described by Tauc's equation [24] and modified by Mott and Davis as follows [25]. By Beer–Lambert's law, the absorption coefficient can be calculated. G is a constant, (HV) represents to the energy of the incident photons, (Topical.) is the optical band gap energy, and the power (m) characterizes electronic transition, where, m = 2 and 1/2 for indirect and direct allowed transition, respectively [24]. In the present work, the optical energy bandgap for the studied films has been calculated using the absorption spectrum fitting (ASF) method. In this method, the optical absorption coefficient expressed as a function of the wavelength of incident photon and Eq. Can be rewritten as [26,27].
Where is the cut-off wavelength corresponding to the optical band gap, c is the light velocity, and h is Planck's constant. Equation can be simplified and rewrite as:
D = [C(hc)m-1 d/2.303].
The equation helps to calculate optical bandgap only by using the absorbance data avoiding the thin film thickness. Thus, the energy band gap can be calculated directly from using the following relation: The value of can be deduced from extrapolating the linear region of (A/)1/m against (-1) curve at (A/ )1/m = 0. The variation of (A/)1/2 with (1/) for direct allowed transition shown in Fig.5. The values for the studied TiO2-(GeO)x films are listed in Table 2. Energy gap values depend on the concentration of GeO2 in the formed film and it is varying from 2.80 to 3.34 eV.
The Refractive index of TiO2-(GeO2)x films was calculated by the following Eq. [28].
The values of the calculated refractive index, n are tabulated in Table 2. The values reveal that the refractive index of all studied films is considerably high. Fig.6 shows the variation of the energy bandgap and refractive index with GeO2 content in the studied films. As seen from Fig.6, the energy bandgap decreased with increasing GeO2, the lower energy gap is responsible for the enhancement of electron injection and transport properties as reported [29, 30]. This enhancement of electron injection and transport in dye-sensitized solar cells leads to the improvement of current density. As a result of the improvement of current density, we expect the fill factor, and the efficiency of the DSSCs increases with increasing GeO2.
Electrical measurements
Figure 7 depicts the photocurrent density-voltage curves (J-V) of DSSCs for the studied films with different GeO2 doping concentrations of TiO2 nanocrystallites photoanodes. We determined the conversion efficiency of the DSSCs by short-circuit current density (Jsc), open circuit potential (Voc), and the intensity of the incident light per unit area (Pin). We calculated the overall conversion efficiency according to Eq, where Jmax and Vmax are the current density and potential at the point of the maximum power respectively. The calculated photovoltaic parameters listed in Table 3. We found that doping with GeO2, short-circuit current density (Jsc) was increased in comparison with undoped sample x = 0 as shown in Fig.8, this is can be attributed to the decreasing of the energy gap of the samples.
On the other hand, at low concentration of doping with GeO2 doping at x = 0.05 and 0.1 open-circuit voltage (Voc) was increased in comparison with undoped sample x = 0 as shown in Fig.8 and at high concentration of doping at x = 0.2 and 0.3 open-circuit voltage (Voc) was decreased. As a result, the efficiency of doped DSSCs devices at low concentrations is higher than that of undoped cells. Due to the decrease of crystal size for doped films, their surface area will be increased. Thus reveals the increasing number of absorbed dyes which increases the number of injected electrons in metal oxide electrodes, leading to an increase of Jsc [31, 32]. As GeO2 content is; 0.1 wt, it could assist as a crystal growth inhibiter that can cause a higher surface area. This is expected to increase the surface area of TiO2 and consequently increases dye loading and photo-generated electrons [20]. In contrast, if the amount of added GeO2 is; 0.1 wt a distinctive decrease in of the binary oxides electrodes was observed. This corresponds to the property of the pure GeO2 electrode [33].
Conclusion
In this study, the effect of (GeO2)x co-doped on dye-sensitized solar cells (DSSCs) TiO2 nanocrystallites photoanodes at very low concentrations : x = 0, 0.05, 0.1, 0.2, and 0.3 wt%) has been studied. Synthesized samples were examined via the X-ray diffraction (XRD) and UV-visible spectroscopy techniques. The photovoltaic characteristics for the prepared samples have been studied by employing J–V measurements. The obtained results have been revealed: XRD pattern showed that the main phase in the undoped sample (x = 0) is a tetragonal one of the rutile - type with P42/mnm space group, while in doped samples the increasing in GeO2 ratio leads to appearance and rise of another orthorhombic phase Ge4Te5 with Pnma space group.
The optical energy gap (Optical) for the studied films increases with increasing GeO2 content and takes values in the range from 2.80 to 3.34 eV, while the optical refractive index decreases. The crystal size of doped films has been decreased as compared with that of pure TiO2. Thus will increase the surface area TiO2 and consequently increases dye loading and photo-generated electrons. J–V photovoltaic characteristics confirms that the DSSCs co-doped with low concentration doping x = 0.05 and 0.1 of GeO2 have high power conversion efficiency , fill factor (FF), and short circuit current density (Jsc) in contrast samples with high concentration doping x = 0.2 and 0.3 of GeO2. Thus it is reasonable to infer that TiO2-(GeO2)x with x =0.05 and 0.1 wt%) films are potential candidates for optoelectrical devices and dye-sensitized solar cells (DSSCs).
Figures captions
- Fig.1. X-ray diffraction patterns for the Tio2-(GeO2)x samples. The peaks associated with another orthorhombic phase Ge4Ti5 are marked with asterisks. The insets show peaks (110) and (101) belonging to the first rutile tetragonal phase with increasing GeO2.
- Fig.2. Observed (symbols) and calculated (line) X-ray diffraction pattern for Tio2-(GeO2)x samples with x =0, 0.1 and 0.2. Vertical bars indicate positions of Bragg reflections for the phases with different structures: first row - tetragonal P42/mnm., second-row - orthorhombic Pnma.
- Fig.3. UV absorption spectra for TiO2-(GeO2)x , (x = 0.0, 0.05, 0.1, 0.2, and 0.3 wt%) thin film samples.
- Fig.4(a-e): Spectral distribution of transmittance T and reflectance R for TiO2-(GeO2)x, (x = 0.0, 0.05, 0.1, 0.2, and 0.3 mol%, respectively) thin film samples.
- Fig.5(a-e): Variation of (A/)1/2 with (-1) for TiO2-(GeO2)x, (x = 0.0, 0.05, 0.1, 0.2, and 0.3 mol%, respectively) thin film samples.
- Fig.6. Variation of energy band gap (Eoptical) and refractive index, n for TiO2-(GeO2)x, (x = 0.0, 0.05, 0.1, 0.2, and 0.3 mol%) thin film samples.
- Fig.7. The photocurrent density-voltage curves (J-V) of DSSCs with different GeO2-doping concentrations of TiO2 photoanodes.
- Fig.8. Dependence of DSSCs characteristics on GeO2 content; (a) Short-circuit current density of photoanode (Jsc), (b) open-circuit voltage (Voc) and (c) solar energy conversion efficiency.
Cite this Page
Crystal Structure, Optical and Electrical Characteristics of Rutile. (2019, Sep 11). Retrieved from https://phdessay.com/crystal-structure-optical-and-electrical-characteristics-of-rutile/
Run a free check or have your essay done for you
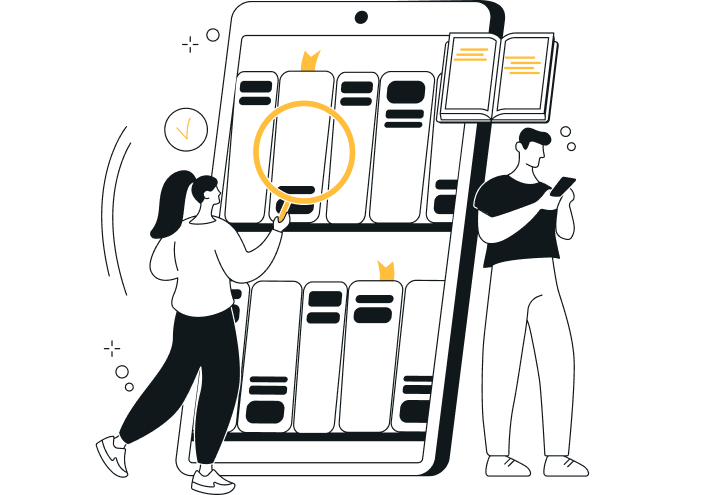