Why We Sleep
WHY WE SLEEP The Functions of Sleep in Humans and Other Mammals J. A. Horne Published by Oxford University Press 1988 Contents CHAPTER 1 Introduction 1. 1Early Sleep Theories 1. 2Daily Sleep and Wakefulness 1. 3Measuring Sleep References CHAPTER 2 Sleep Deprivation 2. 1Problems with Animal Experiments 2. 2Recent Animal Experiments 2. 3Some Problems with Human Experiments 2. 41896 - The First Real Sleep Deprivation Experiment on Humans 2. 5The Longest Study - 264 hours Without Sleep 2. 6Abnormal Behaviour 2. 7The Longest Study With More than One Subject - 205 hours 2. The Walter Reed Experiments 2. 9Motivation and Cerebral Impairment 2. 10Tasks Sensitive to Sleep Deprivation 2. 11Higher Levels of Cerebral Function 2. 12Spare Cerebral Capacity 2. 13Performance Measures Are Too Limited 2. 14Two Types of Sleepiness ? 2. 15Short-Term Sleep Restriction 2. 16Age and Sleep Deprivation 2. 17Does Repeated Deprivation Produce Immunity to Sleep Loss ? 2. 18Can Sleep Deprivation Effects be Sped Up or Slowed Down ? 2. 19Do Long and Short Sleepers Differ in Their Recovery Sleep ? 2. 20Epilepsy 2. 21Other Effects On the Human EEG
References CHAPTER 3 Physiological Effects of Sleep Deprivation 3. 1The First Major Physiological Study - Kleitman, 1923 3. 2The Next Fifty Years 3. 3Body Restitution and Sleep 3. 4Effects on Exercise 3. 5The Control of Body Temperature (Thermoregulation) 3. 6Other Aspects of Homeostasis 3. 7Update on Hormone Changes 3. 8The Immune System 3. 9Conclusions about Sleep Deprivation in Humans References CHAPTER 4 Body Restitution and Sleep 4. 1Tissue Restitution : Protein Turnover and Cell Division 4. 2Factors Influencing Protein Turnover and the Cell Cycle 4. Feeding and Protein Turnover 4. 4Mitosis, Sleep and Physical Activity 4. 5Metabolism During Sleep and the Energy Cost of Restitution 4. 6Cell Energy Charge and Sleep 4. 7Human Growth Hormone Release During Sleep 4. 8Other Hormonal Changes During Human Sleep 4. 9Thyroid Activity and Sleep - Body Versus Brain Restitution 4. 10The Effects of Exercise on Sleep - Background 4. 11Is Body Heating the Key ? 4. 12Conclusions References CHAPTER 5 Waking Awareness, Subsequent Sleep, and Cerebral "Restitution" 5. 1Background 5. 2Influences of Wakefulness on Subsequent Sleep 5. SWS Changes over the Night, and "Models" of SWS 5. 4Brain Work During Wakefulness 5. 5Increased Awareness during Wakefulness and Subsequent Sleep 5. 6Reduced Sensory Stimulation during Wakefulness 5. 7SWS Reductions in Psychiatric Disorders 5. 8SWS and Ageing 5. 9SWS Deprivation 5. 10Brain and Behaviour During SWS 5. 11Cerebral Restitution During SWS ? 5. 12Sleep "Substances" and Immunoen hancement 5. 13Conclusions References CHAPTER 6 Core and Optional Sleep 6. 1Introduction 6. 2Natural Long and Short Sleepers amongst Humans 6. 3Can the Normal Sleeper Adapt to Less Sleep ? . 4Sleep Extension 6. 5Are We Chronically Sleep Deprived ? 6. 6The Circadian Timing of Sleep 6. 7Abnormalities in the Timing of Sleep 6. 8Insomnia 6. 9Stage 2 Sleep 6. 10Conclusions References CHAPTER 7 Sleep in Other Mammals 7. 1Dolphins 7. 2Laboratory vs. Natural Habitats 7. 3Statistical Analyses of Mammalian Sleep 7. 4Sleep - the Immobiliser and Energy Conserver for Small Mammals 7. 5More Energy saving if Sleep develops into a Torpor 7. 6Night versus Day Sleeping Mammals 7. 7Food, Feeding Behaviour and Cerebral Development 7. 8Encephalisation 7. 9Conclusions so Far 7. 0Infancy References CHAPTER 8 REM Sleep 8. 1Perspectives on Dreaming 8. 2Memory, Homeostatic, Sentinel, and Motivational Theories 8. 3Abundance of REM Sleep in Early Life - The Ontogenetic Hypothesis 8. 4Sleep After Increased Learning 8. 5REM Sleep Deprivation in Animals - Background 8. 6REM Sleep Deprivation, Learning and Drive Behaviour 8. 7REM Sleep Deprivation in Humans 8. 8Brain Protein Synthesis and Related Findings 8. 9Conclusions so Far 8. 10Similarities between REM Sleep and Wakefulness 8. 11Keeping Cool 8. 12Keeping Warm 8. 13Increased Heat Production without Shivering . 14Thermoregulation in REM Sleep Reverts to the Foetal Level 8. 15Conclusions About REM Sleep References EPILOGUE Why Do We Sleep ? CHAPTER 1 INTRODUCTION This is a book about the purpose of sleep in mammals, particularly in humans. My approach has been to take a broad biological perspective, looking at sleep in relation to the natural lifestyles and behaviour of mammals, and making what I hope is a series of informed opinions about what sleep means to them, and especially to us. Of course, I do not have the answer to why we sleep, as too much is still unknown.
What I have attempted to do is clear away many misconceptions and try and make some sense of what is left. This book is not meant to be a comprehensive text on sleep, but a selective and personal account giving several hypotheses about a variety of aspects on sleep. Many of my conclusions may well turn out to be wrong, as that is the way of most theories. However, I hope that before they fail they prove to be of use in stimulating other ideas. I have tried to make the book readable, and present my case within an unfurling story about sleep.
Order custom essay Why We Sleep with free plagiarism report
Technicalities have been kept to a minimum, although at times, and of necessity, it goes into some detail. Wherever possible, I have tried to make it understandable, as the book is aimed not only at sleep researchers, but at a readership having more of a passing interest in sleep, with only a basic background in biology and psychology. Little coverage is given to the brain's neurophysiological and neurochemical mechanisms regulating sleep. Whilst they help explain how sleep occurs, the fundamental questions about what they are doing there in the first place, that is the function of sleep, still have to be answered.
Besides, there are already excellent texts describing these mechanisms (e. g. ref. 1). Many people feel that, despite fifty years of research, all we can conclude about the function of sleep is that it overcomes sleepiness, and that the only reliable finding from sleep deprivation experiments is that sleep loss makes us sleepy. Such a forlorn outlook has been partly responsible for many sleep researchers turning away from basic research to the more stimulating field of sleep disorders. Besides, is knowing why we sleep such a vital question after all ?
Employment prospects are far better in the area of sleep disorders, and there is the satisfaction of being able to help or cure many patients. Numerous Sleep Disorders Centres have been established in the United States and Europe over the last decade (alas, not in the UK), and this is by far the greatest growth area in sleep research. Whilst it could rightly be argued that sleep disorders is a far more worthwhile area for sleep research, unfortunately, like the neurophysiological mechanisms of sleep, it still does not tell us much about why we sleep.
Certainly, it has provided valuable information about the neurophysiological mechanisms, and about the association between sleep and breathing (which is not really related to the function of sleep either). This is why the book contains little about sleep disorders. Again, there are already several excellent accounts available (e. g. refs. 2-4). The aim of this book is to show that we have not reached a dead-end in our understanding about the functions of sleep, but rather, that we may have taken too much for granted.
As will be seen, this topic is still an unknown and exciting entity, with many avenues still to follow, and there is much work to be done. Writings about why we sleep date back to before the days of Aristotle. Most couch the purpose of sleep in terms of rest and recovery from the "wear and tear" of wakefulness. One cannot really argue with this idea as it makes so much sense, and besides, we all know that we feel the "worse for wear" without sleep, and so much better after sleep. Nevertheless it is a vague idea - what exactly is recovered ?
This is still a matter for considerable debate, as will be seen throughout the book. It is commonly thought that 7 - 8 hours sleep a night is necessary. This idea is reinforced from many quarters. For example, by the popular press ("you must get your beauty sleep"), and by many GPs. Asking a patient "how are you sleeping ? ", may only be a stock phrase for helping the GP to establish rapport, but it still emphasises the need for a "good night's sleep". The key symptom of insuffient or disturbed sleep is excessive sleepiness in the daytime.
But many insomniacs do not experience this, and a major concern is about "not getting enough sleep", and what may happen to their health as a consequence. However, we probably do not really need the last few hours of a typical night's sleep, and sleep loss is far less harmful than most would think. Most of the theories about the function of sleep concentrate on dreams or dreaming sleep, nowadays called "rapid eye movement" sleep (REM sleep). Few look at the remaining sleep. Many people believe that we only go to sleep for the purpose of dreaming or having REM sleep.
Clearly, dreams are the most enjoyable and noticable part of sleep, but the importance of this sleep is probably overran ted. As will be seen, a large portion of REM sleep is dispensable, without ill-effect. REM sleep only occupies about one quarter of our nightly sleep, and to call the rest of sleep "non-REM" sleep, by describing it in terms of an absence of REM sleep, not only debases the majority of sleep, but overlooks what may losely be described as the "deeper" part of non-REM sleep, called "slow wave sleep" (SWS) in humans.
This form of sleep may well turn out to be the most crucial for us. Nevertheless, despite the fact that no-one really knows what REM sleep does, or whether it is "good" for us, there is concern if it is dimimished. For example, if a sleeping tablet leaves REM sleep unchanged, or even increases it, then this is often seen to be a selling point for the drug, and little concern is usually expressed if the drug impairs or alters non-REM sleep, which by the way, many of these tablets do to a noticeable extent.
This is not meant as a criticism of drug companies, as they have only been following the climate of opinion in sleep research. REM sleep has traditionally been viewed to be essential for the normal functioning of the brain during wakefulness, while non-REM sleep, particularly SWS, is for the rest of the body. Rightly or wrongly so, the old idea of "dualism", the body versus mind controversy in biology has strongly influenced perspectives on sleep. However, as will be seen, this is too simple an explanation, as apart from anything else, the functions of sleep have probably changed with mammalian evolution.
For example, whereas sleep may well provide generalised body tissue repair for the mouse, it is unlikely that this is the case for humans, where sleep seems particularly beneficial to the cerebrum. On the other hand, for us, and perhaps even the mouse, REM sleep may largely be some form of substitute for wakefulness, keeping the brain stimulated without having to awaken the sleeper. Perhaps dreams themselves are just a "cinema of the mind" - the brain's great entertainer to while away the nighttime hours !
However, it is important not to fall into the trap of thinking about each type of sleep in isolation, each having its own distinct function, separate from whatever the other types of sleep are doing. Sleep is a complex process and it is likely that different types of sleep interact with one another to promote a variety of functions, even though one type of sleep may be associated more with one function than another. The last three paragraphs are introductions to most of the key themes of this book, which are developed a little more at the end of the next Section.
Each chapter expands these themes further, and there are summaries at the end of most chapters. Each chapter is a fairly self-contained unit, and does not have to be read in sequence, although this is recommended. A grand summary of all the main themes is given in the last chapter, "Why Do We Sleep", and the reader might like to take a preview of it. 1. 1 Early Sleep Theories Apart from viewing sleep as some sort of recovery process, most of the early theories only looked at the the mechanisms that produce sleep, rather than what exactly sleep does.
For example, believing it to be the result of a build up of some substance in the brain during wakefulness, that is dissipated during sleep. Even Aristotle thought along these lines two thousand years ago, and considered that sleep resulted from warm vapours rising from within the stomach: "The evaporation attendant upon the process of nutrition .. naturally tends to move upward. This explains why fits of drowsiness are especially apt to come after meals. It also follows certain forms of fatigue; for fatigue operates as a solvent, and the dissolved (warm) matter acts like food prior to digestion".
In the last century, with more advancements in the understanding of the brain, heart and vascular system, one school of thought considered sleep to be caused by the "congestion of the brain" by blood. This contrasted with another popular theory at the time, of "cerebral anaemia", due to blood being drawn away from the brain and diverted elsewhere in the body, especially to the gut. Such ideas even led to opposing beliefs about how to induce "better" sleep. Some propounded sleeping without pillows to encourage blood flow to the head, and others encouraged the opposite - use plenty of pillows to drain the blood away. Behavioural" theories were also common in the 19th century, particularly that sleep was due to an absence of external stimulation, with wakefulness only being possible if the organism was constantly stimulated. Take the stimulation away and the animal will fall asleep. To some extent this notion is true, as we can all testify, but it is not the answer. At the turn of the century another behavioural theory became very popular, proposed by a Frenchman, Dr Eduard Claparede.
He considered that sleep was not so much a passive response, but an active process like an instinct, to avoid fatigue occurring - "we sleep not because we are intoxicated or exhausted, but in order to prevent our becoming intoxicated or exhausted". For him, sleep ends when we have had enough. An interesting idea initially, but it has as much depth of understanding as saying that we eat in order to prevent ourselves from starving. The real purpose for eating is to provide nutrients, that undergo complex processes which allow the body to live, grow, and repair itself.
The beginning of the 20th century also produced many of what are termed "humoral" theories, whereby various sleep inducing substances accumulated in the brain. These ranged from known chemicals like lactic acid, carbon dioxide and cholesterol, to the vaguely described "leucomaines" and "urotoxins". Nevertheless, by 1907 some headway began to be made when two French researchers, Drs Rene Legendre and Henri Pieron, claimed to have obtained a substance they called "hypnotoxin" from sleep deprived animals. This gave a large boost to the humoral theories for the next twenty years or so, with much activity by several groups of researchers.
However, success was hard to come by and interest dwindled. That is, until the 1960s, when great headway has since been made into "sleep substances" (see Section 5. 12). In those interim years most of the excitement came from advances in neurophysiology that could be related to sleep, and a spate of different neural "inhibition" theories for sleep appeared. Many had had their early impetus from Pavlov's views on "cortical inhibition" - that sleep originated from a form of blocking within the cerebral hemispheres.
Although Pavlov vehemently dismissed the alternative, of sleep inducing "centres" in more basic parts of the brain below the cortex, these have since been found to exist, and have become the centre of one of the prominent fields of sleep research, especially, after the discovery in the late 1940s, of arousal centres in the reticular formation. Unfortunately though, sleep centres and humoral theories still do not tell us much about the purpose of sleep, in the same way that knowing about centres in the brain that regulate eating behaviour explain little about the purpose of eating.
Hypotheses about the function of sleep have centred on various types of recovery following the wear and tear of wakefulness, and come under the heading of "restorative" theories. In contrast, there are alternatives that reject this standpoint and claim that sleep is non-restorative - simply a form of instinct or "non-behaviour" for keeping us, as well as other mammals, out of harm's way, and occupying the otherwise tedious and unproductive hours of darkness. Through this immobility, sleep will also prevent any waste of energy through needlessly moving about.
Hence sleep is often seen as an "energy conserver". Whilst I believe that these restitutional and instinctive theories have their merits, they seem to fail because each is usually applied universally to all mammals. Why should the functions of sleep for a small nocturnal mammal like the mouse, with a poorly developed cerebral cortex, unable to relax during wakefulness, continually having to forage for food and be on the lookout for predators, be exactly the same as that for humans, who are usually the opposite in all these respects?
One theme I shall be developing in this book is that these three aspects of sleep function - restoration, energy conservation, and as an occupier of time, will alter as the evolutionary scale is ascended, depending on various interrelated circumstances of the mammal, particularly body size, level of cerebral development, amount of relaxed wakefulness and type of diet. Furthermore, for most mammals including ourselves, the functions of sleep may well alter as each night's sleep progresses, initially serving more important purposes, then changing to those of less benefit.
Not only does this idea break with the traditional division of sleep into REM and non-REM sleep, but also means that the last part of sleep may be "superfluous" in many mammals. For humans, this applies to the last two hours or so of the typical eight hours of nightly sleep. This is similar to eating and drinking, where we can easily consume more than we really need, or do with a little less, without any ill-effects, apart from some harmless adjustment of body weight, for example.
My standpoints on sleep are somewhat heretical, and argue against many commonly held ideas. But before entering this controversy, let me provide a little background about some of the more common phenomena of sleep and how they are measured. 1. 2 Daily Sleep and Wakefulness The lives of all mammals are very much influenced by internal biological clocks under the control of centres within the brain that regulate not only the level of alertness over the day, but the timing of sleep, wakefulness, and most other physiological functions.
There is much debate about whether these rhythms come under the control of one, two, or more central clocks. At the moment it is thought that there may be two, one controlling sleep and wakefulness, and the other body temperature and various aspects of general physiology. On the other hand, it is possible that both are part of some less well understood "masterclock". However, assuming there to be two clocks, it seems that neither runs precisely at 24 hours, and the term "circadian", from the latin circa diem ("about a day") has been adopted to describe them.
Human circadian rhythms are inclined to run a little slower than 24 hours, more like 24. 5 hours, but they are restrained to 24 hours through the brain being aware of regular daily events in the environment. Such events are called "zeitgeibers", a german word losely translated as "time giver". For many mammals sunrise and sunset are the main zeitgebers. If the zeitgeibers are removed, for example, by keeping an animal in an artificial environment under constant light, then the body temperature clock "free runs" at its natural period (i. . 24. 5 hours in humans). But in the modern world of electric lighting, our internal clocks can no longer rely on daylight and darkness as a zeitgeiber, and instead, somehow use other regular cues such as mealtimes, and perhaps morning wakening by an alarm clock. Under normal everyday conditions our internal clocks are linked together, with body temperature and most physiological activities increasing during wakefulness and declining during sleep. This is not simply an effect of different levels of physical activity.
For example, if sleep is lost at night and taken in the day instead, as happens in shift work, the temperature rhythm remains the same for several days, still falling at night and rising by day. Then it flattens out, and eventually begins to re-shape itself to rise at night and fall by day. Full adaptation of the temperature rhythm may take two weeks, and until this occurs, with the sleep-wakefulness rhythm completely resynchronised with it, the shiftworker experiences various discomforts such as sleepiness at work, indigestion, loss of appetite and headaches.
These are not harmful, just annoying, and are in effect a worse form of "jet lag", where the timing of sleep and wakefulness is also suddenly shifted in relation to body temperature and local time. Why Nature has given animals these circadian clocks is not exactly clear, and the reason may vary somewhat from species to species. However, most animals are very much at the mercy of daylight and darkness, irrespective of whether they live diurnal or nocturnal lifestyles.
One view of the function of the circadian clocks is that they preempt each part of the day by ensuring that sleep, wakefulness, alertness, and various physiological changes will be at their most suitable levels. Such preempting may be necessary as there is a time lag for these changes to occur, which might be too long if they did not begin until the external event arrived. For example, a warm brain works better than a cool one, but during the sleep period body and brain temperature fall a little. Some time is required for the brain to warm up, and if this did not begin until wakefulness then behaviour could be impaired for a while.
The circadian clock seems to anticipate wakefulness and starts the warm-up process a few hours beforehand, ensuring that the brain is at a good working temperature when wakefulness begins. 1. 3 Measuring Sleep If one simply watches a sleeping mammal, including humans, certain common features are seen: A typical body posture A specific site or nest for this behaviour Physical inactivity A regular daily occurrence influenced by a circadian clock More stimulation is required to rouse the animal than during wakefulness However, advanced mammals like ourselves can feign some of these haracteristics during wakefulness by resting with the eyes shut, and a more accurate method for measuring sleep is needed. Furthermore, as it is tedious to watch an animal sleeping for many hours at a time, some form of automatic recording is desirable. The organ that shows the clearest changes during sleep compared with relaxed wakefulness is the brain, and this is particularly obvious in its electrical activity. Concentrating on the brain in this way is appropriate in other respects, as not only does it contain the control mechanisms of sleep, but of all the body's organs it is for the brain and behaviour (i. . mainly the cerebral cortex) that sleep seems to be the most vital. Monitoring this electrical activity in animals involves surgery and the placing of minute electrodes in the brain and other parts of the head. These are normally connected by flexible wires to a junction box above the cage. This can restrict the animals' movement, and if more freedom is wanted then a minature radio transmitter can be fixed to the head instead. In humans, electrodes are only fixed to the surface of the scalp with a quick-drying sterile glue, easily removed by a solvent.
Wires from the electrodes are plugged into a junction box, and the signals amplified by a machine similar to that used for animals. Such amplifiers are technically very sophisticated as they have to boost the brain's signals by about a million-fold, because the electrical activity of the brain is only at a few millionths of a volt. After amplification, the signals can be written out by mechanical ink pens on paper, or recorded on magnetic tape. The human brain largely consists of the cerebral cortex (sometimes called the "encephalon") surrounding the rest of the brain like the canopy of a mushroom around its stalk.
As the electrodes are located above the cortex, the electrical activity they pick up is that of the cortex, rather than of deeper brain areas. Hence the term "electroencephalography" (EEG for short) is used to describe this technique of scalp recording. The paper write-out is called an "electroencephalogram" (also called the EEG), and the machine containing the amplifiers and pens, as an "electroencephalograph". When electrodes are placed in the cortex itself, as with animals, the electrical activity should strictly be called he "electrocorticogram" (ECoG). However, for simplicity, many people including myself, also refer to it as the EEG, even though this is incorrect. Much of sleep can be assessed from the EEG alone, but for the measurement of REM sleep, additional electrodes have to be placed around the eyes to detect the rapid eye movements, and over muscles in the chin or neck. For reasons that are not understood, in REM sleep these muscles profoundly relax ("tonus" is lost), and this can be used as a further guide to REM sleep.
Although muscles in the rest of the body do not lose their tonus, they are unable to move as there is also a type of paralysis going on during REM sleep that prevents voluntary movement. For many mammals the EEG of REM sleep is very much like that of aroused wakefulness, which is why REM sleep used to be called "paradoxical" sleep - the animal is behaviourally asleep, but the brain seems to be awake. So without knowing about the activities of the eyes and neck muscles, we could easily mistake REM sleep for wakefulness.
For humans the EEG of REM sleep is very much like that of light non-REM sleep (stage 1 sleep), and consequently was once called "stage 1-REM sleep". Again, eye and neck muscle recordings are essential, to separate REM sleep from stage 1 sleep. The EEG consists of waves that can be measured in terms of: AMPLITUDE: The voltage between the peak and the trough of a wave, and measured in millionths of a volt (microvolts - uV). Amplitude rises as consciousness falls - from alert wakefulness, through drowsiness to deep sleep. FREQUENCY: The number of complete waves or cycles occurring in one second, and expressed as "hertz" (hz - cycles per second).
The effective range in the human EEG is from about 0. 5 hz to 25 hz. Generally speaking, frequencies above about 15 hz are "fast waves", and frequencies of under about 3. 5 hz are "slow waves" - these are the waves of slow wave sleep (SWS). Whereas amplitude rises as sleep deepens, frequency falls. With the very advanced mammals, especially apes and humans, the EEG of both wakefulness and sleep is more complex, and enables further specific types of EEG to be identified according to certain frequency bands. These are given greek letters, and going from high to low frequencies the MAIN divisions are as follows (there are some gaps):
BETA - is usually above 15 hz and consists of fast waves of low amplitude (under 10 uV) that occur when the cerebrum is alert or even anxious. ALPHA - is normally the range 8 - 11 hz, and is typical of relaxed wakefulness, and when there is little input to the eyes, especially when they are shut or staring at a blank wall. THETA - is in the range of 3. 5 - 7. 5 hz and it reflects drowsiness and light sleep DELTA - these are the slow waves of SWS, and have the lowest EEG frequency, of under 3. 5 hz. They are of a high amplitude, often over 100 uV, and increase in appearance as sleep becomes "deeper".
There are some other, more transient EEG activities found only during sleep, such as "vertex sharp waves" occuring with theta activity at sleep onset, and spindles and "K complexes" that are most prominent in stage 2 sleep (see Section 6. 9). All these EEG characteristics allow human non-REM sleep to be broken down further, and there are standard reference works for this purpose, one for infants (5) and the other for adults (6), describing in detail the EEG and other characteristics of REM sleep and of the four increasingly "deeper" EEG stages of non-REM sleep - stages 1, 2, 3 and 4 sleep.
However, the staging of non-REM sleep is arbitarily defined and still a matter for debate, particularly in the case of the elderly (7). Nevertheless, this sleep staging is generally accepted. Wakefulness is called stage 0, and is typified by alpha or beta activities. Stage 1 is really a transition stage from wakefulness or drowsiness to true sleep (stage 2 sleep or deeper), and usually only occupies about 5% of the night. Stage 1 is typified by theta activity, a loss of alpha, and often some vertex sharp waves. There is also much "eye rolling", as the eyelids slowly open and shut a few times, with the eyes rolling upwards and downwards.
If one watches someone falling asleep, especially if they are also struggling to remain awake, then these movements of the eyes and eyelids can be clearly seen. The bulk of human sleep, around 45 % of it, is made up of stage 2 sleep, containing a mixture of theta activity, sleep spindles, K complexes and a few delta waves. Stage 3 is more of a transition phase from stage 2 to stage 4, and only constitutes about 7% of sleep in the young adult. It contains 20 - 50 % delta activity of a certain amplitude. When this activity goes beyond 50% then the "deepest" sleep, stage 4, is reached.
This makes up about 13% of sleep in the young adult. SWS is the collective term for stages 3 and 4 sleep, where delta activity increasingly predominates. The EEG characteristics of the various sleep stages are shown in Figure 1. REM sleep occurs regularly throughout sleep in nearly all mammals. The time from the beginning of one episode of REM sleep to the beginning of the following is remarkably regular within any species, and seems to depend on the brain size of that species (8). The larger the brain, the longer this time interval. Whilst in humans it is about 90 minutes, for the rat it is only about 12 minutes.
Interestingly, although REM sleep only makes up a small portion of total sleep in most mammal species, normally about 10 - 15%, humans have roughly double this value. However, for all of them, including humans, this declines with age (Figure 21), and as I have already mentioned, is much more evident in the newborn. REM sleep is discussed in detail in Chapter 8. Figure 1. 1: EEG of Human Sleep Stages Wakefulness shows alpha activity (subject relaxed) and beta activity (alert). Theta activity can be seen in Stage 1 sleep. Stage 2 sleep shows spindles and a K complex.
Note the large slow waves (delta activity) of stage 4, also apparent to some extent in stage 3 sleep. Stages 3 and 4 together are "slow wave sleep" (SWS). The EEG of REM sleep resembles that of stage 1, and contains a mixture of beta and theta activities. To avoid mistaking these two stages, recordings are made of eye movements and chin muscle tonus (see text). Usually, for humans each minute or half-minute of sleep is broken down into the sleep stages, and the results can be plotted out as a "hypnogram". A simplified version is seen in Figure 2, and shows certain key features of sleep: ) A rapid descent to stage 4 sleep soon after sleep onset. 2) A regular 90 minute cycling of REM sleep and other stages. 3) The prevalence of stages 3 and 4 sleep (SWS) in the first cycle, less in the second cycle, and only some stage 3 sleep in the third cycle. SWS is largely confined to the first half of sleep. 4) A greater predominance of REM sleep and stage 2 sleep in the second half of the night. Figure 1. 2: A simplified "hypnogram" of sleep stage changes over the night in young human adults REFERENCES 1. McGinty D. J. , Drucker-Colin R. , Morrison A. & Parmeggiani P-L. (eds) Brain Mechanisms of Sleep, New York: Raven Press (1985). 2. Williams R. L. , & Karacan I. (eds) Sleep Disorders, Diagnosis and Treatment, New York: Wiley and Sons (1978). 3. Chase M. , & Weitzman, E. (eds) Sleep Disorders : Basic and Clinical Research, New York : MTP Press (1983) . 4. Parkes J. D. Sleep and Its Disorders, London : W. B. Saunders Co (1985). 5. Anders T. , Emde R. , & Parmelee, A. A Manual of Standardised Terminology, Techniques and Criteria for Scoring States of Sleep and Wakefulness in the Newborn Infant.
Los Angeles: UCLA Brain Information Service (1971). 6. Rechtschaffen A. & Kales A. A Manual of Standardised Terminology, Techniques and Scoring System of Sleep Stages in Human Subjects. Los Angeles: UCLA Brain Information Service (1968). 7. Webb W. B. & Drebelow L. M. A modified method for scoring slow wave sleep of older subjects. Sleep, 5, 195 - 199 (1982). 8. Zepelin H. , & Rechtschaffen A. Mammalian sleep, longevity and energy metabolism. Brain and Behavioural Evolution, 10, 425 - 470 (1974). CHAPTER 2 SLEEP DEPRIVATION . 1. Problems with Animal Experiments One way of finding out about the functions of sleep is through sleep deprivation, and there have been many such investigations on animals and humans since the turn of the century. The general findings are, that although humans appear to cope fairly well, other mammals tend to come off worse. This does not necessarily mean that humans have different sleep functions to those of animals, but that most of the animal experiments have introduced additional stresses which have been more eventful.
With humans, we can ask for volunteers to go without sleep for a few days, and impress on them that they are free to withdraw whenever then want. Also, these volunteers are carefully looked after, their safety is ensured, and nothing harmful will be allowed to happen to them. However, none of these factors really apply to animals, as for example, we cannot communicate these assurances to them, and so to speak, put their minds at rest and allay apprehension. Their natural lifestyle is totally disrupted, as they are kept awake at times of the day when they expect to sleep, through methods they do not understand and have no control over.
Although sleep deprivation in animals can be given for a longer time than for humans, implying that more interesting findings might be forthcoming, we can still be more confident that the results from human sleep deprivation studies are less affected by additional stresses. The first well-documented experiment of this type on animals was carried out in France during 1894 by a Dr M. de Manaceine, who kept puppies awake for 4-6 days by walking or handling them continually. By the end of this time their body temperatures had fallen by about 4°C, and there was a drop in the number of red blood cells.
Autopsies revealed many small haemorrhages in the cerebral cortex. These findings stimulated much interest and soon led to further studies by other laboratories, also on puppies. Again, falls in body temperature were found, and although changes in the cerebral cortex were also reported, these were more variable and of a different nature to those of Manaceine's puppies. However, few of these experiments used a control group of animals, and it is likely that some of the changes attributed to sleep deprivation may have been due simply to laboratory techniques unrelated to the deprivation itself.
Ideally a control group would consist of littermates allowed to sleep normally, so that comparisons could be made with the deprived animals. Such a method was subsequently used in the substantive studies carried out by an American, Dr Nathaniel Kleitman, in the 1920s. Over the next 40 years he performed many more investigations into sleep in both animals and humans, and for these efforts he is usually regarded as the "father" of sleep research. His great work, "Sleep and Wakefulness" (1), was for many years the textbook on sleep.
In Kleitman's early experiments, puppies were kept awake for 2-7 days, by groups of assistants walking or playing with the animals. This technique was successful for up to 3-4 days of continued wakefulness, with most of the animals feeding and drinking normally. Thereafter though, they would lose all interest in the surroundings. However, when they were compared with littermate control animals that were not sleep deprived, there was no greater fall in body temperature, nor any important change to vital functions.
The only real finding was a confirmation of the earlier reports of a drop in the number of red blood cells in the sleep deprived animals. Examination of the brains of all the animals showed similar and clear abnormalities for both the groups. Whilst the true reasons for these latter effects are unknown, it is likely that the damage was done during autopsy, as the techniques used for preparing the brains for analysis were crude by today's standards. Other sleep deprivation experiments of this era used rabbits, but again, few used control groups. Probably the best known was by another American, Dr W G Crile, who kept rabbits awake for 4-5 days.
A slight rise in body temperature and a slowing of respiration were found, but no fall in red blood cells. Autopsies revealed changes to the liver and adrenal gland, as well as to the cerebral cortex. Although Crile could not explain these findings, again it is likely that the autopsy procedure was to blame. These early studies simply relied on the experimenters' claims that their animals remained awake, and it was not until the 1950s that advances in EEG recording techniques made it possible to measure whether the animal was truly asleep or awake.
So until recent times, about the only way of making sure that the animal remained awake was to keep it continually moving, but this meant that one was now looking at the effects of physical activity plus sleep deprivation. To some extent the influence of the physical activity alone could be "subtracted" by giving the same amount of exercise to a control group allowed to sleep. However, it is possible that exercise interacts with sleep deprivation in a way not found in the control group, as for example, forced exercise when wanting to sleep may be more stressful to a sleep deprived animal than to a refreshed control animal.
This is a problem that Kleitman readily acknowledged, even though he did use control groups. Nevertheless, this questioning about the impact of exercise may be rather theoretical, as it will be remembered that few of the early deprivation experiments which used exercise found any serious abnormality anyway - at least up to seven days wakefulness, although using the limited and rather crude methods for determining an animal's state of health. There is one more of the early sleep deprivation experiments that I must mention, carried out in 1946 by Drs J C R Licklider and M E Bunch, from Harvard and Washington Universities (2).
Their first aim was to determine the least amount of sleep that laboratory rats could survive on, as usually these animals slept around 12 hours a day. Animals were kept awake by forced walking on a treadmill. Very much to the experimenters' credit, a variety of control groups were used. In an initial pilot experiment, animals were divided into four groups : no sleep, normal sleep, 8 hours sleep, and 4 hours sleep. They were kept like this for several weeks, or, as was to be the case for the totally sleep deprived group, until they died.
This usually occurred after 3 - 14 days. To Licklider and Bunch's surprise, the four hour group seemed to survive "indefinitely". The only finding of note was that these animals were extremely irritable and had to be handled with caution. Licklider and Bunch's next experiment, their major one, now looked in more detail at the effects of four hours sleep per day, but this time on young (adolescent) animals, particularly, at their rate of growth and learning ability. Control groups were again used.
Although I will not go into details, suffice to say that these tried to clarify the effects of the exercise itself, and other potential problems. Animals from the experimental and control groups were still growing, and all had access to food all of the time. Measurements were taken for 10 - 18 weeks. However, within a few days from the start, the growth rates of the 4 hour sleepers began to fall behind those of the control groups, and after a further 50 days their body weights just levelled off whilst the others continued to grow.
But according to the investigators the shortened sleepers seemed healthy enough, apart from irritability. Of great interest was that learning in these rats was certainly no worse than that of the control groups - even marginally better. The most sophisticated studies of sleep deprivation using EEG methods also pair together sleep deprived and control animals, so that when sleep onset occurs in the EEG of the sleep deprived animal, it is stimulated into wakefulness. The control animal is similarly stimulated irrespective of whether it is awake or asleep.
Because both animals have similar circadian sleep and wake patterns, the likelihood of sleep is greater at certain times of the day, so whenever the sleep deprived animal is stimulated its partner may also be asleep. Consequently, the control animal also loses some sleep, but only about 20-30% of it, and is certainly not totally sleep deprived. For both animals these laboratory procedures are stressfull, and it is assumed that because one animal has total sleep deprivation plus these stresses, and the other only partial sleep deprivation plus the stresses, any greater effects on the first animal are due to the larger sleep loss.
These sophisticated studies are a great improvement on earlier ones where there was little or no control, but there is still the problem that the sleep deprived animal has a greater disruption to its lifestyle as well as to its sleep. To be stimulated into wakefulness from drowiness or sleep, as is the case for the sleep deprived animal, may be more stressing than to be stimulated whilst already awake, as is the likelihood for the control animal.
Although none of these animal experiments can be perfect, of course, they do have the great advantage over the human studies in that more searching measurements can be done, and autopsies carried out afterwards. Apart from changes in behaviour, one of the best signs of stress in both animals and humans is a marked increase in the output of certain hormones from the adrenal glands, particularly adrenaline and the corticosteroids, with the most notable example of the latter being cortisol.
Adrenaline (otherwise called "epinephrine") is the main hormone produced in the core of the adrenals, the "medulla", whereas the corticosteroids come from the outer layer, the "cortex". Hence the more correct term for these latter hormones is the "adrenocorticosteroids". Cortisol helps the body withstand stress by protecting various tissues against excess damage, for example, by reducing inflammation. It combats shock by making body energy reserves more available, and trying to ensure that the volume of the blood and blood pressure can be maintained.
The number of red cells in the blood falls, as more are switched to a reserve store so that fewer cells would be lost during any bleeding. For reasons that are not clear, it also depresses the immune system. Cortisol can affect the central nervous system and behaviour. Under non-stressful conditions cortisol is released in small amounts throughout the day, and has an obvious circadian rhythm, troughing at the beginning of a mammal's daily sleep period, and peaking around the start of wakefulness.
However, rapid increases can occur within a short while of a stressor occurring, and may be maintained for many days as the adrenal gland can soon grow in size to produce more of the hormone. Eventually though, the gland becomes exhausted and the animal's ability to combat the stressing event fails. Death usually soon ensues. Whilst cortisol helps the organism to endure stress, especially if the animal is helpless and unable to avoid the underlying cause, adrenaline has a more rapid alerting effect, commonly called the "fight or flight" response, designed to help the animal quickly avoid the danger one way or another.
Of the two hormones, adrenaline and cortisol, the sleep deprivation researcher usually prefers to measure the latter, because deprivation generally lasts for days, and this hormone is much easier to measure than is adrenaline. In the 1950s a Canadian, Dr Hans Selye, identified three phases in the cortisol response to stress: alarm, resistance and exhaustion (3), with the last one not usually occurring until many days have elapsed. Although Selye's interpretation is now thought to be too-simple an explanation for what is clearly a complex response, his approach is still reasonable for our purposes.
Whilst injury and illness are major causes for the initial alarm response, it will also occur whenever the body is pushed to extremes, for example during heavy exercise or in very hot or cold environments. More importantly, psychological factors such as apprehension and fear are potent triggers for this hormone. These can substantially add to the effects of more physical stimuli such as injury. Whilst animals usually show rises in cortisol during sleep deprivation, this tends not to be the case with humans.
We can be sleepy, irritable and have a great desire to sleep, but providing we know that no harm will be allowed to come to us, and that we can pull out of the experiment if necessary, then the deprivation will not necessarily be stressful. This suggests that some psychological factor in animals, such as fear, may be influencing their cortisol response to deprivation. It must be borne in mind that illness and tissue damage will also activate the alarm responses. So I cannot be clear about how much of the raised cortisol levels in sleep deprived animals is due to physical illness, fear alone, or fear as a result of the illness. . 2 Recent Animal Experiments The most elaborate sleep deprivation studies ever performed on animals are being run at the Chicago University Sleep Laboratory - a premier sleep laboratory established 25 years ago by Dr. Allan Rechtschaffen, and still under his direction. Kleitman was also at Chicago University, but in another Department. He retired soon after Rechtschaffen's arrival, and his sleep laboratory closed down. Rechtschaffen's pioneering work along so many lines of sleep research has brought him a level of respect from sleep researchers that equals that accorded to Kleitman.
Rechtschaffen and his team began their sleep deprivation experiments on rats in the early 1980s (4 - 8). Two main types of study were performed : (i) total sleep deprivation, and (ii) deprivation of REM sleep only. For each of these there were impressive control procedures using control animals. The centrepiece of this laboratory's equipment was the apparatus for sleep deprivation - a horizontal, circular rotating platform 45 centimeters in diameter, surrounded by shallow water. A vertical barrier divided the platform into halves, allowing a sleep deprived animal to be confined to one side, and a control animal to the other.
The platform could rotate slowly under the barrier. When this occurred, both animals had to move to avoid being gently propelled into the surrounding water. As rats dislike getting wet they would do their best to avoid falling in. Each animal had its EEG continuously monitored by a computer. When sleep was detected in the rat to be deprived of sleep, the platform would promptly rotate, causing the animal to rouse and move along the platform. Its partner, which might be awake or asleep at the time, had to move likewise, and to the same extent.
Generally, control animals lost about 26% of their sleep, compared with about 92% for the experimental group. Although this procedure was indeed stressful to sleep deprived animals, as shown by increases in adrenal gland weights and cortisol secretion, the control animals seemed to experience a similar amount of stress for most of the experimental period, as both these indices rose to similar extents in them as well. For REM sleep deprivation alone, the platform only moved when REM sleep was detected.
The technique was quite effective, as virtually all of REM sleep (99%) could be eliminated, whereas the control partner only lost about 4% of its REM sleep. Although the experimental animals were still able to take most of their non-REM sleep (which makes up about 88% of sleep in the rat), there was some unavoidable loss of the "deeper" form of non-REM sleep. This was a problem of some concern to the investigators, and I will come back to this later. For both types of sleep deprivation, experimental and control animals were under constant light, and food always available.
The environmental temperature was set at what is neutral for the rat. Total sleep deprivation caused general debility, weight loss and death by about the 21st day. The control rats all survived the experience, although they became debilitated and lost weight to some extent. Post mortems were performed on all the animals. Examinations were carried out on the brain, liver, kidneys, spleen, lungs, duodenum, stomach, thyroid and thymus, with the pathologists not being told from which group each animal had come. Surprisingly, no significant differences were found between the two groups for any of these organs.
As the investigators pointed out, one of the remaining possibilities was that death may have been due to undetected biochemical abnormalities. So far though, there is no sign of what, if any, these might be. In the total sleep deprivation procedure, both experimental and control animals ate much more food, whilst also losing weight. However, these effects were far more apparent in the experimental group. Calculations by the investigators on the energy obtained from digested food and from breakdown of the animals' own body tissues, showed a very large rise in the energy usage, to around 2. times the baseline levels for the experimental group, and to 1. 7 times these values for the control group. It seemed that such increases were being used to fuel a large rise in metabolism. Although most of this energy need was coming from voracious eating, particularly in the experimental animals, the fall in body weight showed that the animals' own energy stores were being depleted. But this weight loss was probably not the cause of death in the sleep deprived animals, as at death their weight had only fallen to about 80% of the starting value.
The investigators had shown that starved rats not sleep deprived can still survive at 70% of their original body weight. Sleep deprived animals were digesting their food normally, and there was no sign of diabetes or other illnesses that could account for weight loss and voracious eating. So what was happening to all this energy, and was it all going to fuel increased metabolism? The gland that has a major effect on metabolic rate is the thyroid, but its hormones showed no changes. Could "stress" still have an answer?
There were no noticable differences in the size of the adrenal glands between the two groups for most of the time, from the start of the deprivation until the death of the sleep deprived animal. However, there was a rise in adrenal weight a few days before death. To try and determine the stress responses further, the investigators made more detailed analyses of blood samples from both groups of animals, every few days. Apart from the measurement of cortisol and other blood constituents, assessments were also made of the hormone that controls cortisol release - adrenocorticotrophic hormone (ACTH).
Both the sleep deprived and control groups showed similar rises in these two hormones, with no significant differences between the two groups for either substance. Except of course, for the few days prior to the death of the experimental animal, when there was a greater rise in cortisol. The other blood constituents showed no notable differences between the groups, except that the hormone noradrenaline (norepinephrine) was far higher in the experimental animals. The exact reasons for this were not really known.
This hormone has many interesting actions, with several relating to metabolism and the regulation of heat loss from the body. For example, it limits heat loss from the skin by restricting blood flow to skin capillaries, also, it makes body fat supplies more easily available as an energy source for other tissues. One important role for noradrenaline is to stimulate special heat producing organs called "brown adipose tissue" (BAT or "brown fat") to burn up more energy for heat. However, this tissue is usually only found in infant animals and is gone by adulthood.
It is not known if the rats used in the Chicago studies had any brown fat reserves. I shall cover the role of this tissue in more detail, in Section 8. 13. An important finding with the totally sleep deprived group was that body temperature fell during their last days, and this, together with the earlier increases in feeding and raised metabolism, suggests that something seriously happens to the ability of the animal to conserve its body heat - that is, its thermoregulation becomes impaired. This state of affairs was not so apparent in the control animals.
What seems to be happening, although we cannot be certain, is that soon after sleep deprivation begins, experimental animals increasingly lose body heat, presumably through the skin, and compensate by burning more energy to increase metabolism and create more body heat. More and more food has to be eaten, but even this is not enough, as body energy reserves also have to be sacrificed on this metabolic fire. For the first two weeks, heat production matches heat loss, as body temperature stays normal.
But then there is a deterioration, with heat loss exceeding heat production, and body temperature falls. Although death follows a few days later, it is not yet known whether this is due directly to the collapse of thermoregulation, or just that this collapse is a symptom of something more subtle but nevertheless catastrophic, not yet understood. The control group did not reach this state, as their body temperature never fell, at least up to the time they were killed, when their experimental partners died.
The physical appearances of the sleep deprived animals changed in a characteristic way, apart from the weight loss. After about a week of total sleep deprivation their hair developed a yellowish tinge and became matted. The skin of the tail and paws developed small red inflamed areas that eventually developed into often quite large lesions, very much like ulcers, but containing only a minor infection. These got worse as the deprivation progressed, and also began to appear in the control group, but at a slower rate of development.
Surprisingly, all the animals seemed unconcerned about these sores and paid little attention to them. The lesions were only on the bare skin of the tail and feet, and were not found under the fur. Careful examinations, by specialists in skin diseases, were made of the lesions, and it was concluded that these were not due to wetness, or pressure on the skin - they remain a puzzle. Although there are suspicions that a biochemical change in the skin may be the cause, perhaps even a vitamin deficiency, there is no evidence of this, despite careful chemical analyses.
To see whether the animals were becoming debilitated from infections, blood was analysed to find out if the immune system was functioning normally. Immunology is a highly complex area (see Section 3. 8), and only a few tests could be performed. Nevertheless, such tests would have been discerning enough to pick up anything unusual going on - but nothing remarkable was found. In fact, the remarkable finding was that there was nothing unusual, given the animals' circumstances. What is happening to the sleep deprived rat - so many blanks have been drawn?
All that we apparently have so far is what seems to be a problem with thermoregulation, and the skin lesions - neither of these seemed to be due to the animals getting wet through falling in the water, as this was also investigated. As far as can be seen, little else seems to be going wrong. Rechtschaffen and colleagues are cautious over speculating over their findings. Although they believe that heat loss and thermoregulation lie at the crux of the demise of their animals, they emphasise that more proof is required.
This would, for example, come from careful measurements of metabolic rate, which so far have been difficult to carry out in the sleep deprivation apparatus. Usually, animals would be put inside a calorimiter (a chamber for measuring body heat production), but a sleep deprived animal quickly falls asleep here, and sleep alters metabolic rate. Nevertheless, I would like to concentrate on thermoregulation a little more as I believe it to be a crucial factor. Firstly, let me give some more background, because even the normal and healthy rat has potential problems with its thermoregulation.
Like other small mammals it has a relatively large body surface area in proportion to its weight. Simple geometry shows that as body weight doubles, surface area only increases by about 60%. Keep on doubling size in this way until something of the mass of a human is produced, and the body surface becomes quite small in proportion to its weight. If there is any deterioration in the ability of the body surface to keep heat in, then this will become an increasing problem the smaller the mammal, with less body mass to generate heat in proportion to surface area. Body heat loss is less of a concern to humans than it is to rats, mainly ecause of our relatively large mass in proportion to surface area. But the animal usually has effective countermeasures - physiological and behavioural. Both are mostly aimed at protecting its more exposed body areas, the paws, and especially the large tail. Physiologically, blood flow to the skin is reduced here, and behaviourally, the animal can sit on its tail, or curl up into a ball. Now, it seems to be fairly certain that sleep deprived rats are losing a large amount of body heat, and presumably, a major potential route for this loss is through the tail and paws.
Even though these animals are in a neutral environmental temperature, this is still below that of their body, and body heat can still be lost. Since food intake rises and weight falls as soon as deprivation begins, the apparent increase in heat loss seems to begin immediately. I suspect that a sleepy rat is less aware of this loss, and the animal may "forget" to sit on its tail to conserve body heat. It cannot curl up for long as the inevitable sleep and promp movement of the platform makes it wake up and walk. From what I have seen of sleep deprived rats, their tails are almost always exposed.
If too much heat is being lost here, then one would expect the animal to protect its tail as much as possible - but sleep deprived rats are not doing this. They still have the physiological countermeasure, of reducing the blood flow to the skin of the tail and feet. Whether or not the eventual appearance of ulcers in these areas is related to the problems of thermoregulation, or is just a coincidence, is an open question. Perhaps a prolonged restricted blood supply to the skin of the tail and feet, lasting for a week or so, promotes skin ulcers?
Enough of this speculation of mine, and let us return to some other fascinating findings from the Chicago Group. They had suspicions that the inevetable REM sleep loss during total sleep deprivation might have been a key factor in the deterioration of their animals, as REM sleep deprivaton alone, also led to death. But as the investigators noted, the difficulty with this idea is that death occurred much later during REM sleep deprivation, after an average of 37 days - almost twice as long as for totally sleep deprived animals. REM sleep deprivation produced very similar ffects to total sleep deprivation, including the skin lesions, except that the course of events was spread over a longer time. Stress seemed slightly higher in the REM sleep deprived animals than in their control partners, as cortisol and ACTH levels were somewhat higher, but this was not really apparent until about the week before death. The most dramatic difference was again a large increase in food intake, which began soon after the REM sleep deprivaton started. Calculations of the energy used by the REM sleep deprived animals showed a massive 3. times rise over that of the pre-deprivation levels, against a 1. 9 rise for the control group. Body temperature stayed normal for the first two weeks of REM sleep deprivation and then fell, but always remained stable for the control animals. If REM sleep deprivation was the key to the death of the totally sleep deprived animals, why would REM sleep deprivation alone allow them to survive for twice as long? Maybe REM sleep deprivation is of little relevance after all! Instead, perhaps the fatal factor is not the type of sleep that is lost but the amount, of whatever type.
Returning to my earlier line of thinking - the greater the sleep loss the more the sleepiness, and the greater the impairment to the behavioural countermeasures, etc. Remember, as REM sleep deprivation allows animals mo
Cite this Page
Why We Sleep. (2017, Apr 09). Retrieved from https://phdessay.com/why-we-sleep/
Run a free check or have your essay done for you
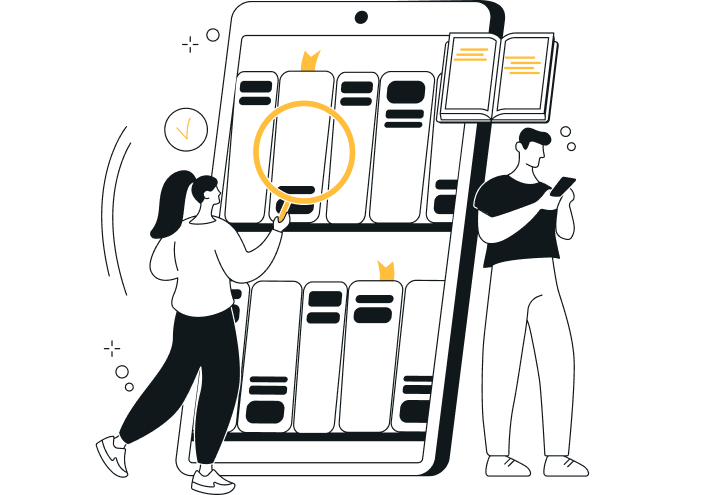