India: Cdm Opportunities and Benefits
IV. India: CDM Opportunities and Benefits Milind Pathak, Leena Srivastava, and Sudhir Sharma* Tata Energy Research Institute, New Delhi Summary More than twenty potential Clean Development Mechanism (CDM) projects in five different sectors are reviewed in this chapter. They include new technologies and fuel-switching options for conventional power generation, applications of renewable technologies for power generation and agricultural activities, and efficiency improvements in the production of cement and iron and steel. All projects advance sustainable development in some form.
Non-climate environmental benefits include improved air and water quality, reduced solid waste, and soil protection. Development benefits include rural electrification, employment opportunities in particular groups, and improvements in industrial efficiency. An analytical tool—the Analytical Hierarchical Process (AHP)—is used to evaluate the benefits of different options— in particular, their consistency with national priorities articulated in the planning process and their environmental and economic development benefits.
Among the abatement opportunities reviewed, there appears to be a very high overlap between projects that are low-cost and projects that are consistent with India’s development priorities. In three of the four sectors for which comparisons can be made, the first and second options ranked by price are also the first and second options when ranked by their non-carbon benefits. This suggests that projects advanced under the CDM would make a significant contribution to India’s own development goals. Planning Priorities and Development Paths
Order custom essay India: Cdm Opportunities and Benefits with free plagiarism report
In India, the planning process takes the form of FiveYear Plans formulated by the Planning Commission. 1 The development objectives laid out for the upcoming period provide a touchstone for assessing the CDM’s potential to address domestic priorities. The objectives stated in the ninth and current Five-Year Plan (1997–2002) reveal a mix of economic, environmental, health, and social goals. They include • promoting agriculture and rural development, • accelerating economic growth while maintaining stable prices, • improving the supply of drinking water and primary health care, containing the population growth rate, • ensuring environmental sustainability of the development process, • empowering women and socially disadvantaged groups, • promoting and developing people’s participatory institutions, and • strengthening efforts to build self-reliance. * The opinions expressed herein are those of the authors and do not necessarily reflect the positions of their institutions. Financing Sustainable Development with the Clean Development Mechanism 51 After independence in 1947, planning was initially geared toward the development of agriculture, with a focus on irrigation and power.
Over time, emphasis has shifted toward energy-intensive sectors and energy generation. The growing importance of the energy sector is evident from its increasing share of total outlay under successive plans, rising from 19 percent of total spending in the first plan to 27 percent in the latest. Energy planning in the Five-Year Plan is supplemented by some medium- and long-term planning by other groups. For example, in 1985, the Advisory Board on Energy drew up plans for the energy sector up to the year 2004–05. Similarly, the Central Electricity Authority has a National Power Plan up to 2011.
The principal objectives outlined in the Ninth Five-Year Plan for the energy sector are to meet the economy’s energy needs through efficient and sustainable use of resources. This will be achieved by developing institutional mechanisms for energy conservation, demand management, research and development (R&D), and regulatory mechanisms. Continuing the effort to promote renewables, the government is aiming for a gradual commercialization of non-conventional energy and increased exploitation of India’s large potential for cogeneration. Planned legislation will enable power producers using these technologies to sell to the grid.
The privatization trend initiated in 1991 will continue. In line with past reforms in energy pricing, tariffs will be rationalized, and the use of “time-of-day” metering and peak-period pricing will be explored for eventual use. Private sector participation will add impetus to the ongoing renovation and modernization program for generating stations. Importing of hydrocarbons and coal fuels for power sectors will be “decanalized”—allowing importers to contract directly with foreign suppliers instead of being forced to obtain imports indirectly through the Indian Oil Corporation.
The agriculture sector still commands substantial attention. Policy plans include augmenting irrigation facilities, intensifying the watershed development program, enabling larger and more timely March 2000 credit flow, setting up crop insurance programs, and conducting R&D into new technologies. More generally, the government hopes to improve the framework for technology transfer and to develop a strong scientific capability domestically. For all sectors, several important obstacles will have to be surmounted to meet development objectives.
These include: a lack of capital, insufficient government resources to meet development objectives, a lack of foreign exchange for ensuring balance of payments sustainability, and inadequate infrastructure. Environmental Pressures and Aims A further sense of India’s environmental priorities can be discerned from current trends. The AsiaPacific Environment Outlook identifies water resources, industrial pollution, urban congestion, land and soil resources, and deforestation as the major environmental issues for India (UNEP, 1997).
Some of these issues overlap with potential CDM projects: Urban Air Pollution. India’s major sources of air pollution are industries (toxic gases), thermal power plants (fly ash and SO2), and motor vehicles (carbon monoxide, lead, and particulate matter) (TERI, 1998b). While particulate levels are high, average SO2 concentrations in these cities are all within World Health Organization (WHO) guidelines (WRI, 1998). Motor vehicles are a major source of urban air pollution, especially for particulates.
Although none of the CDM options reviewed here addresses this issue, projects aimed at improving efficiency and reducing CO2 emission levels from urban transport sources would also do much to improve urban air quality. Solid Waste. Solid waste includes heterogeneous urban household waste and more homogeneous accumulations of industrial, agricultural, and mining wastes. Solid waste in urban areas, mainly municipal and industrial solid wastes, is collected and disposed of by municipal corporations. The other waste is disposed of by the respective industries.
Most Indian cities are generating fast-increasing amounts of municipal waste. Per capita waste http://www. wri. org/ World Resources Institute 52 Financing Sustainable Development with the Clean Development Mechanism generation is growing by 1. 33 percent a year (TERI, 1998c). Indian cities and towns generate an estimated 4,000 tons (t) of municipal wastes every day, creating pressure for land space and generating air pollutants and other problems with their disposal. In 1991, nearly 90 percent of waste was landfilled (EPTRI, 1995). Industrial solid waste is made up of nonhazardous, non-biodegradable waste.
The major generators of industrial waste are: thermal power plants, which produce coal ash, integrated iron and steel mills, which produce blast furnace slag and steel melting slag; nonferrous industries such as aluminum, zinc, and copper, which produce red mud and tailings; and fertilizer and allied industries, which produce gypsum. Overall, the industrial sector generates an estimated 100 million tons (Mt) of nonhazardous solid wastes—with coal ash from thermal power stations accounting for more than 70Mt—and 2 Mt of hazardous waste a year (TERI, 1998b).
Industrial waste poses a serious threat to the environment because its disposal is largely uncontrolled. Dumping it on public land or introducing it into the municipal waste stream is common. The treatment and disposal of hazardous waste is particularly problematic. Chemical processes to destroy hazardous substances and biotechnological processes to detoxify hazardous components are seldom employed. Highly toxic wastes are sometimes destroyed by controlled incineration but more commonly, small quantities of sludge are burned with factory garbage in open pits (Bhoyar et al. 1996). Uncontrolled dumping is less common than secure landfilling. Water Pollution. While municipal sources contribute three quarters of wastewater measured by volume, industrial waste makes up more than half of the total pollution load. Sewage treatment facilities in major cities can often process only 10 percent of the wastewater generated. On average, the treatment capacity in Class I cities can handle only 60 percent of the wastewater collected and less than 15 percent of the total volume of wastewater generated. Average figures for Class II towns indicate about 90 percent of the water supply is polluted, and only 1. 6 March 2000 percent of the polluted wastewater gets treated (CPCB, 1990). Of the total pollution contributed by industrial subsectors, 40 to 45 percent is from processing of industrial chemicals; nearly 40 percent of all organic pollution comes from the food products industry alone (World Bank, 1996). Food products and agrobased industries together contribute 65 to 70 percent of all industrial wastewater in terms of organic load.
Of the 426 large- and medium-sized industries in the region covered by the Ganga Action Plan, 101 do not have adequate pollution control facilities. These include sugar mills, distilleries, leather processing units, and thermal power stations. Small industries, in particular, lack such treatment facilities. Coal mining also degrades water quality in specific areas. Land Use and Deforestation. Deforestation, though less extreme in India than in other countries, is putting rapidly growing pressures on forest resources.
The main causes of deforestation are from conversion to river valley projects (for both power and irrigation purposes) and from legal and illegal harvesting to meet timber and firewood needs (Ravindranath and Somashekhar, 1995). With economic and demographic growth, demand is expected to rise sharply for timber, from 30 Mt a year in 2000 to 43 Mt in 2020, and for firewood, from 185 Mt to 272 Mt (Ravindranath and Somashekhar, 1995). Meeting these demands poses challenges for the Indian forestry sector, quite apart from the carbon implications.
Deforestation creates various problems including damage to soil and water resources as well as loss of biodiversity. To date, India has addressed the issue through a series of Forest Conservation Acts and an Afforestation Program. The main policy thrust is to monitor and protect existing forests and to reduce inherent pressures on forest resources by extending biomass production under management programs that involve local communities. The CDM offers potential to extend or supplement these efforts. Climate Change. Though not currently a priority, compared to pressing non-climate environmental
World Resources Institute http://www. wri. org/ Financing Sustainable Development with the Clean Development Mechanism 53 problems, India could be more at risk than many other countries from changes in temperature and sea level. Models predict an average increase in temperature in India of 2. 3 to 4. 8 oC for the benchmark doubling of CO2 scenario (Lonergan, 1998). Temperatures would rise more in Northern India than in the South. In the North Indian Ocean, under a doubling, the average number of tropical disturbance days could increase from 17 to 29 a year (Haarsma et al. 993); while, without protection, approximately 7 million people would be displaced, and 5,760 km2 of land and 4,200 kilometers (km) of road would be lost (Asthana, 1993). The dominant cost would be the land loss, accounting for 83 percent of all damages. For the same CO2 doubling scenario, a crop-simulation study estimates that wheat yields could decrease between 28 and 68 percent (Rao and Sinha, 1994). Even allowing for adaptation options, agricultural losses could be significant. The loss in farm revenue is estimated at 9 to 25 percent for a temperature rise of 2 to oC (Kumar and Parikh, 1998) Energy Trends Indian energy supply relies heavily on coal use—a trend likely to continue as rising energy demand spurs on new capacity development. 3 Industry is the biggest user of commercial energy. Although only a third of rural households are now electrified, the residential sector will become an important source of demand. Although coal is forecast to dominate, India could support significant amounts of renewable technology capacity—wind, solar, and biomass. Conventional Energy Generation
Vital to the development process, India’s energy sector has been a major beneficiary under the planning system. The public sector outlay for the energy sector in 1996–97 was Rs. 115,000 crores (US$3. 2 billion). Nearly 70 percent has been set aside for power generation. Between 1984 and 1994, final commercial energy consumption grew at a rate of about 5. 6 Figure 4. 1 Sectoral Consumption of Commercial Energy in India, 1994 (Mtoe) 100 90 80 Million tons of oil equivalent 70 60 50 40 30 20 10 0 Agriculture Source: TERI, 1998b. Electricity Natural gas Petroleum products Coal
Industry Residential Transport Others March 2000 World Resources Institute http://www. wri. org/ 54 Financing Sustainable Development with the Clean Development Mechanism Figure 4. 2 Gross Electricity Generation 1950-95 (billion kWh) 400 350 300 250 Nuclear 200 150 100 50 0 1950 Source: MoF, 1996 Billions of kilowatt hours Thermal Hydro 1960 1970 1980 1990 1995 percent a year. Commercial energy fuels now account for 60 percent of overall energy consumption, up from a mere 26 percent in 1950–51. India’s energy-GDP elasticity is fairly high at 0. 9–1. , but on a slightly downward trend. Even with recent growth, per capita commercial energy consumption is low at 0. 48 toe—a mere 6 percent of U. S. levels (IEA, 1998). The industrial sector is the largest consumer of commercial energy, followed by transport. (See Figure 4-1. ) India has an installed capacity of about 87 GW, 65 per cent of it coal-based. The generating capacity of utilities comprises a mix of hydro, thermal, and nuclear plants. After focusing on hydroelectricity projects in the 1960s, thermal capacity has shown a remarkable increase. (See Figure 4-2. The share of hydro capacity has been declining for various reasons, including low public acceptance because of adverse environmental impact and the large-scale displacement of people. The share of nuclear power remained stationary at about 3 percent. Gross power generation from utility capacity has increased from 6 billion kilowatt hours March 2000 (kWh) in 1950–51 to 380 billion kWh in 1995–96— an average growth rate of about 10 percent a year (MoF, 1996). Even at this rate, capacity expansion has been unable to keep pace with demand, due to serious financial constraints.
Shortages remain fairly constant at between 7 and 9 percent, with peak shortages in the range of 15 to 20 percent (MoP, 1996). The situation may worsen in the face of expected demand increases, following economic liberalization policies and national objectives. Less than a third of rural households are electrified. New capacity of 12 GW will be required every year under the next three Five-Year Plans, and power demand by 2020 is estimated at 385 GW, requiring investment of at least US$380 billion in the power sector alone (TERI, 1998b). Coal would remain the dominant fuel for power generation.
Business-as-usual projections for the demand and supply of coal and other primary energy are presented in Table 4-1. Projected increases in carbon emissions from the energy sector are shown in Table 4-2. World Resources Institute http://www. wri. org/ Financing Sustainable Development with the Clean Development Mechanism 55 Renewables India’s renewable energy program can be traced to the first oil shock in the early 1970s. Renewable energy technologies have since been developed and implemented in India for power generation, heating, cooling, lighting, pumping, cooking devices, and as alternative fuels for surface transport.
About 1,050 MW of power generating capacity—7 percent of the total power capacity added during the Eighth Five-Year Plan—was made up of non-conventional energy sources (GoI, 1997). A significant amount came from grid-connected wind-farms, where capacity rose from 47 MW in 1992 to 900 MW in 1997. Even so, renewables comprise less than 2 percent of total installed capacity. Renewable energy sources offer a significant potential to extend energy supply without relying on carbon-intensive fuels and to allow for decentralized electricity generation.
The Ministry for Nonconventional Energy Sources (MNES) has adopted a three-pronged approach for its implementation strategy for renewables: (1) providing budgetary support for R&D and demonstration projects, (2) extending institutional finance through the Indian Renewable Energy Development Association (IREDA) for private sector participation and bilateral and multilateral projects, and (3) promoting private investment through various fiscal incentives such as tax holidays. The MNES has also announced Table 4-2 Table 4-1
Demand and Supply Forecasts for Primary Energy Under 1995-96 Business as Usual Source of Estimates TERI Official Demand Availability Demand Availability Demand Production Demand Production 1995? 96 300 290 325 n. a 2001? 02 Coal (Mt) 387 367 440 346 2006? 07 494 474 557 473 TERI Official Petroleum Products (Mt) 67 103 141 54 97 136 n. a 113 155 n. a 113 156 Natural Gas (billion cubic m3) TERI Demand n. a 42? 56 32 Availability n. a 54? 83 n. a n. a. Not available; TERI Tata Energy Research Institute. Source. TERI, 1998d. certain targets to be achieved in the Ninth Plan.
These include • 3,000 MW of additional power from renewables, • installation of 10,000 PV water pumping systems, • solar water-heating systems for 500,000 households, Projected CO2 and CH4 Emissions in the Baseline (Tg) CO2 emissions (Tg) Energy sector Forestry/land-use sector Total CH4 emissions (in CO2 GWP equivalents, Tg) Enteric Fermentationa Manure Managementb Ricec 1990 532 2 534 143 19 85 247 781 2000 973 29 1,002 154 21 96 270 1,272 2010 1,555 77 1,632 168 22 101 292 1,924 2020 2,308 __ (2,308)d 183 23 108 314 (2,622)d
Total (CH4 only, Tg) Total (CO2 and CH4, Tg) a. The emission projections are made by the Central Leather Research Institute (CLRI), based on animal population growth rate for 1981-87. b. The emission from manure management are also based on same animal population growth rate. c. National Physical Laboratory (NPL) estimates. d. Totals exclude forestry/land use sector. Source: TERI, 1998d. March 2000 World Resources Institute http://www. wri. org/ 56 Financing Sustainable Development with the Clean Development Mechanism 500,000 m2 of solar collectors in industrial/ commercial establishments, • additional small hydro capacity of up to 15 MW (MNES, 1998). Besides new renewable technologies, decentralized bioenergy plans also offer significant potential to meet energy needs in rural areas (Ravindranath and Hall, 1995). projects cover the spectrum of potential CDM activities, including improvements in industrial efficiency, power generation using waste materials, and changes in agricultural practices. Such diversity is consistent with using the AIJ phase as a learning period.
Formed to recommend and evaluate projects, and consisting of representatives from government and nongovernmental organizations (NGOs), the “Task Force on AIJ” continues to assess new proposals. Here, we review 22 separate projects in five different sectors. (see Table 4-4. ) These options form the basis of a comparative ranking using analytical techniques. Typically, these options lead to an improvement in energy efficiency and resource conservation and introduce advanced technologies, so laying the foundation for long-term sustainable development.
Mitigation options in the power sector include clean coal technologies and renewables. Options such as bagasse-based cogeneration and combined cycle plants are already profitable and generate fewer emissions per kWh of electricity than conventional generation. Similarly, small hydro-, wind-, and biomass-based power, though more expensive than conventional coal-based plants, provides significant abatement opportunities. Renewable options for power and irrigation in the agriculture sector are also considered, as are opportunities in the iron, steel, and cement sectors.
Three main criteria governed the choice of these options: their consistency with national development priorities; the relatively high level of energy India’s Greenhouse Gas Emissions Despite initiatives to promote clean energy, the expansion of conventional energy generation, particularly coal-based generation, implies a rapidly growing emissions profile for India. Under business as usual, energy sector CO2 emissions in 2010 may be three times greater than in 1990 (TERI, 1998d). 4 Table 4-2 also illustrates CO2 emissions from the forestry sector and CH4 from the agriculture sector.
Though relatively small in magnitude, forestry and land use emissions are expected to grow rapidly. Agricultural emissions of CH4, accounting for 32 percent of the present global warming potential from these sources, are expected to grow more slowly. Changing development paths now might prevent greenhouse gas (GHG) emissions from reaching such levels (Reid and Goldemberg, 1997). CDM finance would strengthen that trend. Potential CDM Options India already has five CDM-type projects in place under the pilot version of the CDM—Activities Implemented Jointly (AIJ). (See Table 4-3. ) The Table 4-3
Pilot Phase AIJ Projects Endorsed by the Government of India Project name 1 2 3 4 5 Direct Reduced Iron (DRI) Energy recovering from waste gas and liquid Integrated Agricultural Demand Side Management DESI Power: biomass gasification Tamarin Orchard Agroforestry for Dry Land Location Gujarat Gujarat Andhra Pradesh 20 sites Karnataka Investor Japan Japan World Bank Netherlands United States Host M/s Essar, Gujarat, India IPCL Plant at Vadodara, Gujarat, India Andhra Pradesh State Electricity Board, India DESI Power, Development Alternatives, India ADAT, Bagepalli, Karnataka, India March 2000 World Resources Institute ttp://www. wri. org/ Financing Sustainable Development with the Clean Development Mechanism 57 Table 4-4 Potential CDM Projects Sector Conventional power generation Base-case technology Pulverized coal subcritical boilers for all cases Mitigation options Pulverized coal supercritical boilers Atmospheric fluidized bed combustion Pressurized fluidized bed combustion Integrated gasification combined cycle Combined cycle gas turbines? natural gas? based Cogeneration? bagasse? based Renewables for power generation Traditional thermal power generation using domestic coal Small hydro Wind farm Photovoltaic? ased power generation Biomass-based power generation Renewables for agriculture Small-scale diesel pumpsets Agro-waste? based gasifier Wood-waste? based gasifier Wind shallow pump Wind deep pump PV pump Iron and steel Integrated steel plant with wetquenching option Wet quenching Open hearth furnace Conventional electric arc furnace Conventional (normal) casting Cement Non-precalciner kilns Non-preheater kilns Direct reduction process Dry quenching Basic oxygen furnace Ultra-high power electric arc furnace Continuous casting Dry precalciner kilns Dry suspension preheater kilns onsumption in the base activity; and the relatively large GHG reduction potential offered by the abatement technology. (For a detailed assessment of each option, see Appendix 4A. ) Conventional Power Generation Under business as usual, coal would remain India’s predominant fuel for power generation, in view of the large reserves and the high cost of alternatives. To keep up with demand, capacity will have to grow by 12 GW a year for the next 15 years. Improvement in coal generation is certainly possible.
Average gross conversion efficiency of coal thermal power stations is 28 percent, and average net efficiency about 25 percent (CEA, 1990). A variety of new technologies are available to improve energy efficiency from conventional fuel sources, mostly coal. In addition to reducing CO2 emissions per unit of output, these technologies could significantly reduce—or eliminate—particulates, SO2, and NOx releases. The introduction of such technologies would also constitute “leapfrogging” for the Indian power sector, allowing http://www. wri. org/ March 2000 World Resources Institute 8 Financing Sustainable Development with the Clean Development Mechanism plants to benefit directly from technologies developed elsewhere. Over time, a growing familiarity with such technologies may facilitate further transfer and even domestic production. Two technologies imply fuel switching away from coal to natural gas and bagasse, respectively. The first option uses natural gas in a combined-cycle technology to attain efficiency of 50 percent or more—higher than any coal option. Cobenefits would be commensurately higher than for coal options, too.
Under the bagasse-cogeneration option, sugar mills would develop a capacity to burn bagasse, a byproduct of sugar refining. Social benefits under this option range from additional income for farmers and sugar mill owners to increased employment opportunities. Even without a credit for carbon reductions, this option is profitable but could be greatly extended with further financing. Iron and Steel India’s iron and steel sector has grown substantially since the industry’s de-licensing in 1991. Total production of finished steel was 17 Mt in 1994–95, with domestic demand projected to rise to 31 Mt by 2001–02. Such high growth is driven by large investments in infrastructure, growth in the transportation sector, and increasing demand for consumer durables. 6 Per capita consumption of only 22 kg—among the lowest in the world—leaves significant room for growth (TERI, 1998a). The private sector is developing 8 Mt of new capacity. The iron and steel industry, where energy accounts for 30 percent of production costs, is India’s largest industrial consumer of energy. However, energy consumption per unit of output is high compared to many other countries—about twice as high as in the old industrial countries nd higher than in the main developing-country competitors (TERI, 1998a). This is for several reasons. Processing the low-grade ores used by the Indian industry takes more energy per unit of output. Most plants in India still use the dated wet quenching method of coke preparation. Modern dry coke quenching improves the coke quality and reduces energy consumption and dust emissions. Efforts are being made to reduce energy consumption by importing good quality coking coal, developing heat energy recovery systems, phasing out the open hearth furnaces for steel making, and increasing the industry’s continuous casting capacity.
Potential CDM activities for the iron and steel sector are listed in Table 4-4. These are based on introducing current technology into all or part of the steel-making process. Such technology will lower CO2 emissions and simultaneously raise the efficiency of steel making in India. Unfortunately, lack of adequate data prevents estimation of carbon price ($/tC), and so improvements are expressed only in terms of reductions per ton of finished steel. Renewable Energy Sources and Technologies The growth of conventional new energy capacity has slowed during the Eighth Five-Year Plan.
Projects in power generation, coal mining, and oil and gas exploration—which require long gestation periods and significant levels of investment and financial restructuring—are lagging behind demand. Consequently, there is even greater opportunity for renewable energy technologies to meet capacity demands. Renewable options were considered for both power generation and for power supply and irrigation in the agriculture sector. Four power-generation options are examined: small hydro, wind farms, biomass gasifiers, and photovoltaics.
Decentralized power may create new jobs by enhancing local energy supply and expanding maintenance needs for power generation (e. g. , through energy plantations for biomass) or by promoting economic growth generally. Increased demand for biomass gasifiers and photovoltaics would also create job opportunities in the manufacturing sector. As renewable technologies, adverse environmental impacts are minimized or non-existent. Similar benefits pertain to renewable technologies for the agricultural sector. Cement India is the fourth largest producer of cement after China, Japan, and the United States.
The cement industry is one of the six most energy-intensive http://www. wri. org/ March 2000 World Resources Institute Financing Sustainable Development with the Clean Development Mechanism 59 industries in India (TERI, 1998a). Cement is manufactured in India using dry, semidry, or wet processes. The current trend favors the dry manufacturing process, which consumes less thermal energy than the wet process, even though it uses more electricity (CMA, 1996; CMA, various years). The cement industry is a continuous process industry where power cuts not only disrupt production but can entail costly damage to equipment.
To maintain uninterrupted cement production, the use of captive power by cement industry is increasing— 27 percent of the total power supply is currently from captive power compared to 16 percent at the start of the decade (CMA, 1997). Two particular abatement options are reviewed here: the introduction of dry precalciner kilns and dry suspension preheater kilns. The first technology allows 85 to 90 percent of the necessary pretreatment (“calcination,” of the “meal” input) to take place at a lower temperature than used in the main kiln, a significant energy saving relative to current technology.
The second technology uses in a preheater kiln waste heat recovered from the main kiln. in India—could support a variety of mitigation projects, from direct carbon sequestration to biomass growth for energy use that would displace coal burning. The ALGAS-India study reviewed a number of alternative forestry sector projects that would mitigate carbon (TERI, 1998d). (See Table 4-5. ) Besides helping to meet growing demands for timber, firewood and electricity, such activities would confer other benefits. Newly afforested areas would reduce present levels of soil erosion and water loss on degraded ands and would enhance biodiversity. Afforestation would also raise grass productivity, extending grazing capacity (Shailajaha et al. , 1994). Large-scale afforestation and reforestation activities would also yield employment opportunities, particularly among rural, low-income groups, through planting, harvesting, and processing of biomass and by supplying non-timber forest products. Different projects offer a different mix of carbon and other benefits. Natural Regeneration would focus primarily on carbon sequestration and would provide a high level of ecological benefits.
Community woodlots would sequester less carbon directly but would meet local demands for biomass as a building material and fuel source. In so doing, they would reduce the burden on women of retrieving firewood and other biomass. Plantations and agroforestry might offer more employment opportunities than other options. Several forestry options have been proposed to USIJI for consideration as AIJ projects. These include a bioelectricity project that would generate Land Use and Forestry Sector
In addition to projects in the industrial and energy sectors, CDM options in the forestry sector offer a mix of carbon and sustainable development benefits. Degraded land, estimated at between 66 and 130 million hectares (ha)—20 to 40 percent of total area Table 4-5 Potential CDM Projects in the Forestry Sector Primary area (Mha) 16 9 13 24 Carbon pool at the end of 40 years (t/ha) 77 171 138 83 Total carbon sequestered (Tg) 1,194 1,611 1,735 2,006 Mitigation option Natural regeneration Enhanced regeneration Marginally degraded level Totally degraded land Private land (agroforestry) Source: TERI (1998d).
Cost (1990 US$/tC) 6 33 40 15 March 2000 World Resources Institute http://www. wri. org/ 60 Financing Sustainable Development with the Clean Development Mechanism electricity from biomass grown on degraded land, a teak plantation for carbon sequestration, and a tamarind agroforestry project, already approved by the Indian Task Force on AIJ, which would provide fruit, halt land degradation and desertification, and sequester carbon (Ravindranath et al. , 1998). All these activities offer sustainable development benefits and are consistent with the priorities of specific Indian ministries.
Though questions persist about which, if any, types of forestry project will be permitted under the CDM, these projects indicate a carbon and sustainable development potential every bit as significant as, and often less costly than, energy and industrial sector projects. Table 4-6 Development Criteria Used to Evaluate Projects Main category Feasibility Subcategory - Consistency with government policy - Socioeconomic development - Project risk Nonclimate environmental benefits Development - Resource conservation - Decrease in pollution loading - Health - Employment generation - Value added - Rural development
Prioritizing CDM options Which of the above options are best depends on one’s perspective. Developed-country investors will be mainly concerned about a project’s abatement cost, financial risk, and feasibility. India will be more concerned about a project’s development and environmental benefits and their consistency with national priorities. Different parties to a transaction will weigh project characteristics differently, suggesting that developed- and developing-country participants would rank overall projects differently. To explore how projects meet national priorities and which, if any, would atisfy all parties, projects were assessed both on the basis of their development benefits and their carbon-abatement cost. While assessing abatement cost is relatively straightforward, evaluating development benefits requires comparison of different types of impact—improvement in air quality, say, against the benefits of rural electrification or employment generation. Inevitably, the assessment of cobenefits is somewhat subjective. However, a model can be used to make the evaluation framework transparent and to explore sensitivities.
Such a model—an Analytical Hierarchical Process (AHP)—was used here. (See Appendix 4B for details. ) Projects were evaluated against nine different criteria that seek to capture India’s development priorities. 7 (See Table 4-6. ) Researchers and governMarch 2000 ment officials were then polled on the importance of different development criteria allowing weights to be placed on these different cobenefits. Scoring each project against these weighted criteria created an approximate assessment of the overall development potential.
The ranking procedure was conducted on a sectoral basis for four of the sectors reviewed above. Projects were ranked both by carbon-abatement cost alone and by a measure of overall development benefits. Table 4-7 illustrates how the two rankings compare. For three of the four sectors for which comparisons can be made, the two highest ranked options, based on cost of carbon offset, are also the two highest ranked options, based on their cobenefits. This suggests a high degree of overlap between projects that would be given priority by carbon-focused investors and projects that are in India’s best interest.
Only the renewables for power generation category shows a mismatch in the first-choice as perceived by market criteria alone and the wider criteria that make up the AHP scores. Also in conventional power generation and renewables for agriculture, some of the lower rankings are different. Conclusions For the abatement options reviewed, there seems to be a high degree of overlap between projects available at lowest cost and projects most consistent with national priorities and offering the most to http://www. wri. org/ World Resources Institute
Financing Sustainable Development with the Clean Development Mechanism 61 Table 4-7 Summary of Sector Rankings and Carbon Price Predicted abatement cost (U. S. dollars/tC removed) Conventional Power Generation Bagasse-based cogeneration Combined cycle generation (natural gas) Atmospheric fluidized bed combustion Pressurized fluidized bed combustion Pulverized coal super-critical boilers Integrated gasification combined cycle –244 –133 7 47 96 96 1 2 3 4 5 5 1 2 5 4 6 3 Ranking by abatement cost Ranking by overall development benefits (base-case weights) Project
Renewables for Power Generation Small hydro Biomass power Wind farm Photovoltaic 29 134 216 1,306 1 2 3 4 2 1 3 4 Renewables for Agriculture Wood-waste gasifier Agro-waste gasifier Wind well (shallow) Wind well (deep) Photovoltaic pump 169 177 298 329 6,333 1 2 3 4 5 1 2 5 4 3 Cement, Iron, and Steel Manufacture Dry suspension preheater kiln Dry precalciner kiln 7 214 1 2 1 2 March 2000 World Resources Institute http://www. wri. org/ 62 Financing Sustainable Development with the Clean Development Mechanism India in terms of development and environment cobenefits.
This paper also demonstrates a useful methodology for evaluating the sustainable development potential of projects offering different types of cobenefit. Inevitably, weighing projects that generate different and unquantifiable cobenefits will be somewhat subjective. The advantage of the ranking (AHP) technique used here is that it creates a transparent framework for handling subjective views on a project’s worth. Over time, the weights given to different types of benefit will have to be constantly updated to reflect the latest preferences manifest in the political process.
This study has presented a preliminary prioritization of CDM projects for India. The current application of that technique could be improved or extended in several ways. Ideally, all projects could be compared together, instead of merely within sectors. This would let policymakers know which sectors should be emphasized before deciding which projects within a sector make most sense. Adding projects and sectors would allow for a more comprehensive evaluation. In particular, including land use and forestry options, despite their current status in the negotiations, would be helpful. The transport sector is another potential area for financing.
Urban air pollution and congestion have been identified as key environmental problems directly traceable to the many inefficient motor vehicles on the road. The CDM could be used to help finance new transport initiatives or to improve engine technologies. Finally, more details on the projects themselves would allow for more accurate evaluation. For example, the health benefits of cleaner coal technologies are sensitive to the precise location of the plant to be modified. Even at this stage, however, it is clear that candidate projects in India could simultaneously make progress on the dual goals set out for the CDM.
Ultimately, it is up to India to decide which projects to put forward for CDM investment. Project evaluation for carbon as well as non-carbon benefits should be further developed to maximize this overlap. March 2000 Notes 1. 2 3 4 This section draws on TERI (1998d). Class I cities have a population of 100,000 or greater; Class II cities have populations of between 50,000 and 100,000. This section draws on TERI (1998b). These are CO2 emissions from the energy sector in a baseline scenario for 1990–2020 assuming a 12 percent discount rate. They were calculated using the MARKAL model.
This is based on moderate estimates of growth of GDP (5 percent to 6 percent a year) Appraisal of the Credit Rating Information Services of India Ltd. (CRISIL). In the formal analysis and rankings shown later in Table 4-7, some recognition was also given to the carbon-abatement cost. This however, amounted to only 7. 5 percent of a project’s ranking and did not alter the overall rankings. 5 6 7 References Asthana (1993) Impacts of Greenhouse Gas Induced Sea-level Rise on Islands and Coasts of India. New Delhi: Department of Environment and Forests, Jawaharlal Nehru University.
Bhoyar, R. V. , Titus, S. K. , Bhide, A. D. , Khanna, P. (1996) Municipal and industrial Solid Waste Management in India. Journal of Indian Association of Environmental Management, 23: 53-64. CEA (1990) Public Electric Supply, All India Statistics, General Review 1986-87. New Delhi: Central Electricity Authority, Government of India. CMA (1996) Indian Cement Industry (Statistics) 1995-96. New Delhi: Cement Manufacturers’ Association. CMA (1997) 36th Annual Report 1996-97. New Delhi: Cement Manufacturers’ Association. CMA (Undated) Various Issues. Indian Cement Industry (Statistics).
New Delhi: Cement Manufacturers’ Association. Confederation of Indian Industries (1996) Environmental Business Opportunities in India: A Guide. New Delhi: CII. CPCB (1990) Status of Water Supply and Wastewater Collection, Treatment and Disposal in Class I Cities—1988. New Delhi: Central Pollution Control Board. EPTRI (1995) Status of Solid Waste Disposal in Metropolis. Hyderabad: Environment Protection Training and Research Institute. Draft. GoI (1997) Annual Plan 1996/97. New Delhi: Planning Commission, Government of India. Haarsma, R. J. , Mitchell, J. F. B. , Senior, C. A. 1993) Tropical Disturances in GCM. Climate Dynamics, Vol. 8, pp. 247257. World Resources Institute http://www. wri. org/ Financing Sustainable Development with the Clean Development Mechanism 63 IEA (1998) Key World Energy Statistics form the IEA, International Energy Agency, Paris, Cedex 15 Kumar, K. S. Kavi, Parikh, J. (1998) Climate Change Impacts on Indian Agriculture: The Ricardian Approach. In Dinar et al. edited Measuring the Impact of Climate Change on Indian Agriculture. World Bank Techical Paper No. 402. Washington, DC: The World Bank. Lonergan (1998) Climate Warming and India. In Dinar et al. dited Measuring the Impact of Climate Change on Indian Agriculture. World Bank Techical Paper No. 402. Washington, DC: The World Bank. MoF (1996) Economic Survey 1995/96. New Delhi: Ministry of Finance, Government of India. MNES (1998) Annual Report 1997/98. New Delhi: Ministry of Non-convential Energy, Government of India. MoF (1996) Economic Survey, 1995/96. New Delhi, Ministry of Finance, Government of India. MoP (1996) Annual Report 1995/96. New Delhi: Ministry of Power, Government of India. Rao, D. G. , and Sinha, S. K. (1994) Impact of Climate Change on Stimulated Wheat Production in India. In C. Rosenzweig and A.
Iglesias edited Implications of Climate Change for International Agriculture: Crop Modelling Study. Washington, DC: United States Environment Protection Agency. Ravindranath, N. and B. Somashekhar (1995) “Potential and Economics of Forestry Options for Carbon Sequestration in India,” Biomass and Bioenergy, Vol. 8, No. 5, pp. 323-336. Ravindranath, N. and D. Hall (1995) Biomass, energy and environment, A developing country perspective from India, Oxford University Press, Oxford. Ravindranath, N, A. Meili and R. Anita (1998) AIJ in the NonEnergy Sector in India:Opportunities and Concerns, Prepared for the U.
S. EPA Climate Policy and Program Division, November. Reid, W. and J. Goldemberg (1997) “Are Developing Countries Already Doing as Much as Industrialized Countries to Slow Climate Change? ” WRI Climate Note. Washington D. C: World Resources Institute. Shailajaha, R, N Ravindranath, H. Somashekar and K Jagadish (1994) “Biomass generation in mixed tree plantations,” Energy and Sustainable Development , Vol. 1, pp. 52-55. TERI (1996) Survey of Slag Utilization in India. New Delhi: TERI. TERI Project Report No. 95EE51. TERI (1998a) Activities Implemented Jointly in India. New Delhi: TERI. TERI Project Report No. 7GW54, pp. 100. TERI (1998b) TEDDY 1998/99. New Delhi: TERI. Draft. TERI (1998c) Looking Back to Think Ahead: GREEN India 2047. New Delhi: TERI. TERI (1998d) India National Report on Asia Least-cost Greenhouse Gas Abatement Strategy (ALGAS). New Delhi: TERI. TERI Project Report No. 95GW52. Draft: June 1998. TERI (1998e) Development of a Framework for Electricity Tariffs in Gujarat. Electrowatt Engineering Services Ltd. in Association with Tata Energy Research Institute. New Delhi: TERI. ADB T. A. No. 2739-IND. Draft Final Report: February 1998. TERI (1998f) Greenhouse Gas Mitigation Study for Southern India.
TERI Project Report No. 95BO51. New Delhi: TERI. Draft: June 1998. UNEP (1997) Asia Pacific Environment Outlook. Bangkok, Thailand: United Nations Environment Program, Environment Assessment Program for Asia and the Pacific. Washington, DC: World Bank, South Asia Regional Office. World Bank (1996) India’s Environment—Taking Stock of Plans, Programs and Priorities. World Resources Institute in collaboration with the United Nations Environment Programme, the United Nations Development Programme, and the World Bank (1998) World Resources Report 1998-99. New York: Oxford University Press.
March 2000 World Resources Institute http://www. wri. org/ 64 Appendix 4A Clean Development Mechanism Options, by Sector Appendix 4A March 2000 World Resources Institute http://www. wri. org/ Table 4A-1 Conventional Power Sector Pulverized coal subcritical boilers Base-case technology. Over the last 50 years, unit size has gradually increased to more than 500 MW. Efficiency rating of a large steam-fired plant is 36 percent or higher. Pulverized coal supercritical boilers Pulverized supercritical boilers are not manufactured in India. However, a single 500 MW unit has been externally financed.
Efficiency is above 38 percent. Atmospheric fluidized bed combustion Combustion of coal under fluidized conditions at atmospheric pressure, with the heat liberated by combustion transferred to the fluid in the water wall to generate steam. Operating efficiencies range from 35 to 38 percent. Pressurized fluidized bed combustion A combined cycle technology for coal-based generation. The conventional combustion chamber of the gas turbine is replaced by a pressurized fluidized bed combustor. The products of combustion pass through a hot gas cleanup system before entering the turbine.
Exhaust gas is utilized in a downstream turbine. Gross efficiencies are 40 to 42 percent. 47 Financing Sustainable Development with the Clean Development Mechanism Parameters Option Incremental costeffectiveness (US$/t CO2 reduction) Reduction in air pollution Base Case 96 7 Base Case Substantially reduced SO2 and CO Complete elimination of particulates. SO2 reductions of 70 to 95 percent; NOx reductions of 50 to 80 percent. Solid waste increases but includes gypsum? a usable byproduct. Neutral Complete elimination of particulates. SO2 reductions of 80 to 95 percent; NOx reductions of 50 to 80 percent.
Solid waste increases but includes gypsum? a usable byproduct. Neutral Reduction in other pollutants Other environmental benefits What social benefits result? (e. g. , poverty alleviation, rural development) Note: US$1 = Rs 40 Base Case Reduced solid waste Base Case Neutral Base Case Neutral Sale of gypsum generates additional income and may create jobs. Sale of gypsum generates additional income and may create jobs. March 2000 World Resources Institute http://www. wri. org/ Appendix 4A Table 4A-1 Conventional Power Sector (continued) Integrated gasification combined cycle A gasification plant converts coal nto a combustible and purified gas, which is passed to a gas turbine. Exhaust gases are captured and generate steam. For second-generation technologies, efficiencies are expected to be 45 to 50 percent. Combined cycle generation based on Natural gas Natural gas combined cycle plants use the waste heat in a heat recovery steam generator (HRSG), generating additional power. Efficiency is 50 percent or higher. Bagasse-based cogeneration Bagasse-fired cogeneration would lead to high efficiency in sugar mills and provide a large capacity, state-of-the-art, off-season fuel supply.
It would require retrofitting existing boilers and installing cogeneration equipment and advanced pollution control equipment. Plant operating efficiency is as high as 80 percent Parameters Option Financing Sustainable Development with the Clean Development Mechanism Carbon Benefits Incremental cost effectiveness (US$/t CO2 reduction) Environmental Benefits Reduction in air pollution Complete elimination of particulates. SO2 reduction of 90 to 99 percent; NOx reduction of 60 to 90 percent. Bottom ash is generated.
Complete elimination of SO2 and particulates; substantial reduction of NOx No solid waste generation Eliminates air pollution. 96 ? 133 ? 244 Reduction in other pollutants (e. g. , solid wastes) Other environmental benefits (e. g. , biodiversity protection, protection of ecosystems) Solid waste is reduced because bagasse is used for power generation. By displacing coal-based power generation, indirect benefits arise from reduced pollution loading from mining and displaced power generation. Neutral Land requirement is substantially reduced. Social Benefits What social benefits result? (e. . , poverty alleviation, rural development) Note: US$1 = Rs 40 Neutral Neutral Additional income generation for both farmers and sugar mill owners. Additional employment generation. 65 66 March 2000 World Resources Institute http://www. wri. org/ Appendix 4A Table 4A-2 Renewables for Power Generation Parameters Description of option Small hydro Small hydro units can take advantage of small discharges from perennial streams and even height differentials in large irrigation systems in the plains. Installed capacity ranges from 3 MW to 15 MW. This is a centralized power-generation option.
Wind farm A wind farm is made up of gridconnected wind electric generators (usually 200kW and above). Although wind generators can be used for both centralized and decentralized power generation, the largest installations to date have been connected to a grid. They mainly employ horizontal axis wind turbines. Grid-connected generators range from 100 to 300 kW range. Units of 0. 5 to 1 MW should soon be available. Biomass power In a gasifier, solid fuel is converted by a series of thermochemical processes into a gaseous fuel that can be combusted. This technology can be used in gridconnected or stand-alone applications.
More experimentation and demonstration needs to be done with small-scale gasification technology (100 to 500 kW). This is a decentralized option. Photovoltaic Solar PV systems convert sunlight into electricity through a semiconductor electronic process. Although the option is for decentralized power generation, large-scale utility options are also possible. The high cost of PV is a major obstacle to its diffusion. Further, although the modules are highly reliable, overall system reliability is constrained by poor performance of balance systems. Costs for this technology will fall with learning. 1,306
Financing Sustainable Development with the Clean Development Mechanism Carbon Benefits Incremental cost effectiveness (US$/t CO2 reduction) Environmental Benefits Air pollution (e. g. , SO2, NOx, TSP, Ozone, CO) No air pollution No air pollution About 70 to 80 percent of the fossil fuel is replaced by biomass, reducing emissions of particulates, SO2 and NOx, otherwise emitted Solid waste generation is minimized as solid waste in the form of briquettes is used as fuel. Enhances local energy security. No air pollution 29 216 134 Reduction in other pollutants (e. g. , solid wastes) Other environmental benefits (e. . , biodiversity protection, protection of ecosystems) None None None Small hydro avoids the problems of large dams such as submergence of productive and/or forest land, loss of biodiversity, displacement of people. Generates local employment, rural development, and other related economic activities. Enhances local energy security. Enhances local energy security. What social benefits result? (e. g. , poverty alleviation, rural development) Creates employment for both skilled and semiskilled workers. Creates local employment opportunities and activities related to energy plantation.
Further, as power generation is decentralized it may lead to regional development of economic and industrial activities. The technology is totally indigenous, so employment in manufacturing may also increase due to the increased utilization of gasifiers. Unlike other options, it is highly capital intensive. But, rapid commercialization might create jobs in manufacturing, installation and maintenance activities. Note: US $1 = Rs 40 March 2000 World Resources Institute http://www. wri. org/ Appendix 4A Table 4A-3 Renewables for Agriculture Parameters Description of option Agro-waste? based gasifiers
Gasifiers fueled by agricultural wastes. The compatibility between gas and engine needs to be resolved. Also capacity building is required in system operation and maintenance. Wood-waste? based gasifiers As previous but fueled by wood wastes Wind shallow well Windmills can be used to pump water in either deep or shallow wells. In India, wind pumps are usually mechanical rather than electric. The technology needs to be further developed to overcome current design problems. Often the same design systems have to be used for different site conditions. Also, there is a lack of proper technical backup. Wind deep well
As previous but with deeper well PV pump Photovoltaics can be used for water pumping and minor irrigation in regions with shallow water tables. Two types of pump are available: surface centrifugal pumps and deep well submersible pumps. Sizes range from 300 Wp to 2,250 Wp. This technology requires significant development. Present constraints consist of low electricity prices for the agriculture sector and the high capital cost. 6,333 Financing Sustainable Development with the Clean Development Mechanism Carbon Benefits Incremental cost effectiveness (US$/t CO2 reduction) Environmental Benefits Reduction in air pollution (e. . , SO2, NOx, TSP, Ozone, CO) 177 169 298 329 Would substantially reduce diesel use and thus particulate and CO emissions. Reductions in SO2 would depend on the quality of fuel used, while NOx reduction would depend on combustion temperature. Both the latter can be controlled to substantially reduce overall pollution Solid waste is significantly reduced through briquetting of solid wastes and more complete combustion. This option would involve significant saving of fossil fuels as well as prevent deforestation. Further, health hazards would be greatly reduced. Same as agrowaste gasifiers
Reduction in air pollutants occurs through displacement of pumpsets using convention fossil fuels (diesel). Same as wind shallow well Reduction in air pollutants occurs through displacement of pumpsets using convention fossil fuels (diesel). Reduction in other pollutants (e. g. , solid wastes) Other environmental benefits (e. g. , biodiversity protection, protection of ecosystems) Same as agrowaste gasifiers Oil sludge from diesel units is displaced. Noise pollution is substantially reduced. Conserves water by using better water management techniques. This in turn leads to soil conservation by reducing top soil erosion.
Same as wind shallow well Oil sludge from diesel units is displaced. Noise pollution is substantially reduced. Conserves water by using better watermanagement techniques. This in turn leads to soil conservation by reducing top soil erosion. Benefits are lower than in the windpump cases. Neutral Same as agrowaste gasifiers Same as wind shallow well Social Benefits What social benefits result? (e. g. , poverty alleviation, rural development) Note: US$1 = Rs 40. Rural development from advanced technology adoption in the agriculture sector and a cleaner environment. Same as agrowaste gasifiers Neutral Same as wind shallow well 7 68 March 2000 World Resources Institute http://www. wri. org/ Appendix 4A Table 4A-4 Iron and Steel Direct reduction process [DRP] Iron ore is directly reduced to iron by using coal or natural gas as fuel before the resulting ? sponge iron? is converted to steel in an electric arc furnace (EAF) adopting ultra-high power furnace technology (UHF). Dry quenching + BF(HP) + TRT + BOF + CC [DQ] Dry quenching of coke using nitrogen gas. Waste heat is recovered from the nitrogen gas to generate power for internal use in the plant. In the ironmaking process, a highpressure blast furnace with a top recovery turbine (TRT) is used.
The remaining waste heat is used for process heat application. The steel-making process uses a basic oxygen furnace (BOF), which uses oxygen to convert pig iron to steel instead of fuel oil as in the open hearth furnace (OHF). The molten steel is cast in continuous caster (CC). Basic oxygen furnace [BOF] Hot metal from the blast furnace is converted directly to steel by blowing oxygen into the metal, thus reducing its carbon content below 1 percent. This technology is applicable only in steel manufacturing in integrated steel plants. It is not commercially available in India.
Ultra-high power electric arc furnace (UHPEAF) An electric arc furnace capable of operating at temperatures of up to 3500oC. This technology is used in manufacturing steel, primarily in sponge iron plants and mini-steel plants that use only scrap as feedstock. However, EAFs have other applications in the abrasives, refractory, and electrochemical industries. This technology also has to be imported. Continuous casting [CC] Molten steel is directly cast to finished steel, totally bypassing the intermediate stage of crude steel manufacture where a substantial amount of energy is wasted in the finishing.
This process is applicable to steel manufacturing in both integrated steel plants and mini-steel plants. Parameters Description of option Financing Sustainable Development with the Clean Development Mechanism Relevant base-case comparison Carbon Benefits Financial cost (1990 US$) Carbon dioxide reduction (Kg CO2/t of iron and steel) Integrated steel plant technology with the wet quenching (WQ) option, blast furnace (BF), open hearth furnace (OHF), reheating furnace (RHF), and normal casting (NC). 230 to 415/ t finished steel (tfs) 457. 6 Kg/tfs
Wet quenching (WQ) Open hearth furnace option with blast furnace (BF), and open hearth furnace (OHF), reheating furnace (RHF), and normal casting (NC). Conventional electric arc furnace Conventional casting 692 to 831 / tfs (without TRT). 57. 6 Kg/tfs 20. 4 M to 40. 9 M. 182. 7 Kg/tfs 42. 6 M to 53. 2 M 1,100 Kg/tfs 95. 9 M. 1,675. 7 Kg/tfs March 2000 World Resources Institute http://www. wri. org/ Appendix 4A Table 4A-4 Iron and Steel (continued) Direct reduction process [DRP] Dry quenching + BF(HP) + TRT + BOF + CC [DQ] Basic oxygen furnace [BOF] Ultra-high power electric arc furnace (UHP-EAF) Continuous casting [CC] Parameters
Environmental Benefits Reduction in air pollution (e. g. , SO2, NOx, TSP, Ozone, CO) Pollution loading Substantial reduction in depends on fuel use. pollution, especially Nonetheless, a particulates substantial reduction in pollution occurs as a result of minimizing operating steps Reduced solid waste No solid waste generated With oxygen replacing fuel oil, all pollutants are substantially reduced. Approximately a twothirds reduction in all pollutants. All pollutants are reduced (as no reheating is required). Financing Sustainable Development with the Clean Development Mechanism Reduction in other pollutants (e. g. , solid wastes)
No solid waste generated As base case Solid waste generation is substantially minimized, as are requirements for cooling water and fuel oil. Indirect benefits from not using cooling water, fuel oil and coal. Other environmental benefits (e. g. , biodiversity protection, protection of ecosystems) Social Benefits What social benefits result? (e. g. , poverty alleviation, rural development) Both water and land requirements are less. No water is used, and environmental hazards are substantially reduced. Substantial. Not only is the waste heat recovery very high, but waste gases from BOF can also be used as fuels in other parts of the plant.
As base case This technology leads to a trade-off: while capital costs are relatively low, the high economies of scale seen in large integrated steel plants cannot be sustained. As base case As base case As base case Indirect benefits as health hazards are minimized. Tfs tons of finished steel. ; M million. Note: US $1 = Rs 40 69 70 March 2000 World Resources Institute http://www. wri. org/ Appendix 4A Table 4-5 Cement Parameters Description of option Dry precalciner kilns Precalcination is a process of treating the raw meal before feeding it to the main kiln.
In the precalcination process, the preheated raw meal is heated at a lower temperature than that in the main kiln. The amount of calcination achieved is between 85 and 90 percent. This technology is applicable only in dry process cement manufacturing. For this case, the base technology is non-precalciner kiln. 214 Dry-Suspension Preheater Kilns Hot gases leaving the main kiln are between 900 and 1100oC and carry a substantial quantity of waste heat. This waste heat is recovered in high-efficiency suspension cyclone preheaters. This technology is available only in dry process cement manufacturing.
For this case, the base case is no preheater kiln. Financing Sustainable Development with the Clean Development Mechanism Carbon Benefits Incremental cost effectiveness (US$/t CO2 reduction)a Environmental Benefits Reduction in air pollution (e. g. , SO2, NOx, TSP, Ozone, CO) Reduction in other pollutants (e. g. , solid wastes) Other environmental benefits (e. g. , biodiversity protection, protection of ecosystems) Social Benefits What social benefits result? (e. g. , poverty alleviation, rural development) 7 Reduction in pollutants as energy consumption is substantially reduced.
As relevant base case As relevant base case A reduction in pollution is likely because of the low energy consumption during the calcination process. As relevant base case As relevant base case As relevant base case As relevant base case Notes:Plant capacity is 3,000 tons per day; and the plant works 310 days a year. US $1 = Rs 40 Financing Sustainable Development with the Clean Development Mechanism 71 Appendix 4B Ranking Projects Offering Different Types of Noncarbon Benefits Projects are difficult to rank because many important criteria are unquantifiable and non-comparable.
How, for example, should a project that leads to airquality improvements be compared with a project that generates jobs? To tackle this problem, an Analytical Hierarchical Process (AHP) technique is used. AHP provides a tool for scoring and weighting a mitigation option’s unquantifiable attributes. Though providing no single answers, the AHP results reveal which projects will be preferred under different criteria and how rankings would change if certain criteria were weighted differently. AHP lends transparency and structure to project evaluation and decisionmaking.
To assess and set priorities for mitigation options, we have used the Hierarchical Preference Analysis model (HIPRE) developed by the Systems Analysis Laboratory of the Helsinki University of Technology. The process involves ranking a project by four criteria: cost-effectiveness, feasibility and consistency with national priorities, non-climate benefits, and development benefits. Aggregating these for each project allows project comparisons. Priorities were developed for each sector reviewed. CDM options are evaluated against the four following criteria, three of them with further subcriteria: Incremental cost effectiveness: the incremental cost per unit of CO2 reduction. • Feasibility: including consistency with existing government policy; perceived risks from both the investors’ and the host country’s points of view; and consistency with national priorities, namely, social and economic development. • Other
Cite this Page
India: Cdm Opportunities and Benefits. (2017, Dec 11). Retrieved from https://phdessay.com/india-cdm-opportunities-and-benefits/
Run a free check or have your essay done for you
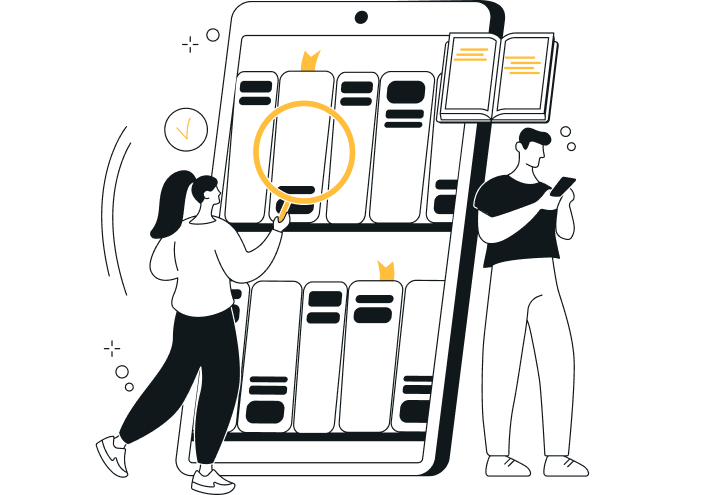