Aerofoil Design Xflr5
ABSTRACT
Airfoil is the main part of the airplane which contributes the lift required by the airplane to fly in the air. By varying the wing’s area and the angle of attack, different lift can be created and can be used to fly the airplane. This program named XFLR5 is used for determining the airfoil characteristics.
It will help them to save a lot of time on computing the equations and on the trial and error method. The program can also be used as basic tool to show the users what will happen when some variables like Reynolds number, the AOA of the wings and the speed of the aircraft are changed and how it will affect the results and lift generated. This project report deals with the analysis of widely used airfoils in the modern aircrafts. In this analysis, the lift, drag, pressure profiles and their coefficients of the airfoils are been calculated using xflr5 program.
Order custom essay Aerofoil Design Xflr5 with free plagiarism report
These are obtained in the form of graphical representation by feeding data such as Reynolds number, Mach number, Angle of Attack and dimensions of the airfoils to the program. The results such as maximum lift coefficient, stalling angle, maximum lift to drag ratio and such parameters are obtained and are been compared with each other.
INTRODUCTION
Airfoils are the primary shapes which allow streamlined flow over it when it is driven through air. These shapes do not produce much drag compared to other shapes as so it is used in all aircraft structural parts which is subjected to airflow. Basically airfoils can be divided into many types by slightly varying their thickness, length, camber area and so on without after the shape.
In this analysis we deal with airfoil shapes known as NACA airfoils. The NACA airfoils are just shapes of airfoils used for aircraft wings, which is developed by the National Advisory Committee for Aeronautics (NACA). The shape of the NACA airfoils is determined using a series of digits followed by the word "NACA. " The series is divided using digits from 1-8. We only deal with the 4-digit series of airfoils in this analysis. The 4 digit airfoils we are going to discuss in this analysis are 0004, 0008, 0012, 0016, 0020, 0312, 1312, 2312, 3312, 2112, 2512 and 2712.
In this 4 digit series, 1st digit denotes the maximum camber as percentage of the chord, 2nd digit denotes the distance of maximum camber from the airfoil leading edge in tens of percent's of the chord and this last 3rd and 4th digits denotes maximum thickness of the airfoil as percent of the chord. The key features we are going to discuss about in this analysis are the effects of Angle of Attack, Reynolds number, Mach number, Camber and Thickness. The basic aerodynamic design is to obtain maximum lift and minimum drag which will be expressed in terms of coefficient of lift (Cl) and coefficient of drag (Cd).
Using this program known as XFLR5, we are going to compare the characteristics of all the airfoils. As the operating valves such as Angle of Attack, Mach number, Reynolds number are to be fed in to the program and this will give us the required graph of the parameters such as Cl, Cd, alpha, Cp and so on. Using these results achieved from the program, it will be easier to find out the airfoil with highest Cl at various angles of attack, which has got highest stalling angle and the airfoil with best glide ratio and so on.
OBJECTIVES
This report is basically done to find out airfoils with maximum lift to drag ratio by comparing many airfoils each other and allowing their camber area, thickness and the location of the camber to deviate to the small percentage of the chord. By doing this, we can achieve a best airfoil design that can be used in live aircrafts.
LITERATURE REVIEW
Introduction:
If an aircraft has to fly, force has to be counter balanced by the weight as it must be opposite to its magnitude. This force is known as lift. Lift plays a major factor on the airplane flight.
The main plane (wings) generates more lift as it is the important load carrying member. * The aerofoil sections have been started to get developed in late 1800’s. In 1884 H. F. Phillips with the help of wind tunnel, tested and made series of airfoils. At the same time Otto Lilienthal with the help of the bird’s wings, designed airfoil shapes. It was found that the key factor is that the wing curvature or camber. The early airplanes were biplanes because, the airfoils were thin and cambered as shown in figure 2. 1. Figure 2. 1: Hanson http://www. biplanefun. om/assets/Image/Hanson_215217_001. jpg Nowadays these types of aircrafts are not used, as all airfoils are made by trial and error method. Airfoils used in 1920’s (NACA). ( http://www. desktop. aero/appliedaero/airfoils1/images/sections1. gif) Figure 2. 2: Ancient development in airfoils For the project, the NACA 4 digit airfoil format will be use for the program which is generating the airfoil’s shape and the thin airfoil theory will be use for computing the important parameters of the wing.
Terminology for Airfoil and Airplane:
Basic parts of an airfoil that must be known before getting in to the chapter of NACA 4 digit series. The diagram below describes the parts of an airfoil from which series are determined. Figure 2. 3: Airfoil Terminology airfoil (http://www. copters. com/aero/pictures/Fig_2-10. gif) Figure 2. 5: Plane Axes (http://www. allstar. fiu. edu/aero/images/fig25. gif)
Definitions: The line drawn midway between the upper and lower surfaces of the airfoil is known as mean camber.
The straight line joining the leading and trailing edges of the airfoil is known as chord. Leading edge is the front of the airfoil. Trailing edge is the end of the airfoil. The angle between the chord and the line representing the relative airflow is known as the angle of attack (AOA). Camber of an airfoil is the upper and lower surfaces curvature. The airfoil’s thickness and amount of lift the airfoil will generate is determined by the cambered area. When the term upper camber is used, it is referring to the upper surface of the airfoil. When the term lower camber is used, it is referring to the lower surface of the airfoil.
Span is the distance from a tip of the wing to the other tip of the wing.
The Four Forces on the Airplane Figure 2. 6: Forces on an airplane (http://anjungsainssmkss. files. wordpress. com/2011/09/plane_forces. jpg) The four main forces that maintain the aircraft in steady flight and helps fly are Lift, Drag, Weight and Thrust. Thrust is a forward pulling which is generated by the engine(powerplant). Thrust opposes the drag. Thrust depends upon the power of the engine as high power engines produce more thrust and the type of the engines with respect to their mounted location.
Drag is a force which opposes the thrust as when the aircraft moves in high speed the air passes over and below the surface produce drag. It is a resistive force. The weight is a force of an airplane which is acting downwards due to the force of gravity. This force pulls down on the plane and opposes the lift force. This force mainly depends upon the weight of the aircraft and so it depends upon how much we load and the total weight we use to build the aircraft. Lift is an important force in the aircraft which tends the aircraft to fly.
It is generated as aircraft moves at high speed and is generated below the airfoil surface of the main plane. At this time, there will be low pressure acting over the wing and high pressure under the wing. The four forces act on any airplanes in flight and are interconnected. In order for the airplane to take off, lift must overcome weight and thrust must overcome drag. During landing, thrust must be reduced below the level of drag and lift must be reduced below the weight of the airplane.
Theory of Lift:
Lift is an important force as it sustains an airplane in ir and enables it to manoeuvre. The theory of lift has been debated for a long time and currently still debating. However this project is not about debating the theory of lift but to design the airfoil. Therefore to cover the theory of lift, the 2 more popular explanations which are the Newton’s Law and the Bernoulli’s Principle will be discuss briefly. * Principles of Physics such as the principle of conservation of momentum, the principle of the conservation of mass and the principle of the conservation of energy are the basic principles to be known and to be considered to understand lift force.
The principle of the conservation of momentum is covered by Newton’s Law. And the principle of the conservation of energy is covered Bernoulli Principles. Both can be use to explain the basics principles on how lift is form and are compatible with each other. In the following explanations, air is assumed as an ideal fluid and therefore 3 major assumptions about the physical properties of the airflow are made: * The airflow over a wing is incompressible. The airflow is inviscid which means the viscosity of air is approaching zero. The airflow around the wing is steady.
Newton’s Law Explanation:
Lift is generated by a wing as it moves through the air at an angle of attack. This will turn the airflow downward and the wing itself will have the force acting in an upward direction. In order to make use of the Newton’s Law, we need to know a theory known as the Coanda Effect. Coanda Effect stated “A moving stream of fluid in contact with a curved surface will tend to follow the curvature of the surface rather than continue travelling in a straight line. ” This states that, when the air hits the wing when it is subjected through air, the angle changes the wing dimension as it is tilted to an extent.
At this stage air do not reflect back as it stays over the wing. From Coanda Effect, we know that the wing is changing the direction of the airflow and also changing the velocity. Since lift is a force, according to Newton’s 2nd law of motion: Force = mass x acceleration (F=ma) Acceleration is a change in velocity with respect to time and force will be: Force = mass x (change in velocity / change in time) Therefore a change in velocity will generate a force and a force will cause a change in velocity.
Velocity has both a magnitude called speed and a direction is associated with it. It is a vector quantity. Therefore by changing the direction of the airflow, the wing is also causing an increase in velocity which results in an acceleration. This change in velocity also generates a reaction force on the wing acting in an upwards direction. * This principle of Newton’s 3rd law which states “Every action has an equal and opposite reaction” helps to explain the nature of lift. The reaction force experienced by the wing is the total reaction. Figure 2. : Forces on an airfoil (http://www. free-online-private-pilot-ground-school. com/images/forces_airfoil. gif) * 1. vii Bernoulli’s Principle: Bernoulli’s Principle can be referred to as the law of conservation of energy and the total energy in a moving mass of fluid consists of potential energy, kinetic energy and the fluid’s pressure energy. It states that sum of all energies result in a constant value for the steady flow of an ideal fluid. Bernoulli’s Principle shows that as the velocity of a fluid flow changes, the pressure will change as well.
This means that when the fluid’s velocity increases, the pressure will decrease and vice versa. Using this principle of pressure variation with velocity change, lift force developed by the wing can be accurately predicted in the same way as Newton’s Law. Figure 2. 8: Example of Bernoulli’s Principle (http://images. rcuniverse. com/magazine/reviews/455/bernoulli. jpg) Using Bernoulli’s Principle, the kinetic energy of the air will be highest where the pressure energy is the lowest and lowest where the pressure energy is the highest.
This will result in an increase of the airflow for the upper surface of the wing and decreases for the lower surface of the wing. Therefore there will be a lower pressure on the upper surface of the wing and a higher pressure on the lower surface of the wing. This will result in a force acting in an upwards direction. Using equation: Force = Pressure x Area The lift force will be proportional to the pressure differential across the wing multiplied by the wing’s surface area. For Bernoulli’s Principle, it does not provide any explanation on why the airflow is faster on the top surface of the wing.
The Newton’s Law also did not explain why the air deflects downward. Both theories only explain how lift is created. * 1. viiiThe Factors affecting Lift: Lift is the main source of force which enables the airplanes to fly. In order to generate enough lift, several factors have to be taken into consideration as they affect the amount of lift the airplane can generate. The general equation for lift is Lift = lift coefficient x {(air density x velocity2)/2} x wing area Where the lift coefficient is given by: Lift coefficient = 2 x pi x (AOA – calculated AOA at zero lift) Therefore the factors affecting lift are: The Angle of Attack (AOA).
- The airfoil shape.
- Airspeed.
- Wing size.
- Air density.
The air density depends on the height which the airplane is flying. As the height of the airplane decreases, the air density will increase which will also result in higher lift generated. Therefore to generate the same amount of lift when the air density increases, the velocity of the airplane must increase. The Angle of Attack (AOA) of the airplane’s wing which is denoted by the Greek letter alpha ?. AOA is the angle between the oncoming air or relative wind and a reference line on the wing which in this case is the chord.
It is one of the important parameters for handling and designing of a plane as a typical wing only has a limited range of angles of attack As the AOA increases, the lift generated by the airfoil is also increased. However, once the AOA reaches a certain angle known as the Critical Angle, the airplane will stall as the airflow will separate from the upper surface, resulting in a loss of lift which means the airplane will stall. This will result in cavitations and lose in lift force. It will also produce drag force as shown in figure 2. 9. Figure 2. 9: Diagram of how AOA affects the air flow (http://www. ero-mechanic. com/wp-content/uploads/2008/12/2-8-283x300. jpg) The lift depends upon the airfoil and the force the air flows over it. Thus when airflow is more, lift produced is maximum. Comparing a cambered airfoil and a symmetric airfoil, a cambered airfoil produced more lift than a symmetric airfoil. Even a flat plate subjected to the air will produce lift. Thus the airfoil should be designed in such a way that it produces high lift to drag ratio. * 1. ix NACA 4 series: In the 1930s, the National Advisory Committee for Aeronautics (NACA) developed several sets of airfoils and camber lines.
The “Characteristics of 78 Related Airfoil Sections from Tests in the Variable Density Wind Tunnel” report was published by the NACA. In this report, the authors discovered that the airfoils that are successful had many similarities. The two primary variables that affected the airfoil shapes are the slope of the airfoil mean camber line and the thickness distribution above and below the line. Many of this airfoil shapes have been in use as tails and wings of airplanes over the years. The first family of airfoils designed using this approached is the NACA 4 series.
In the NACA 4 series, the 1st digit indicates the maximum camber (m) in percentage of the chord, the 2nd digit indicates the position of the maximum camber (p) in tenths of cord and the last 2 digits provide the maximum thickness (t) of the airfoil in percentage of chord. For example, the NACA 2412 airfoil will have a maximum camber of 2% located at 40% from the leading edge with maximum thickness of 12% cord. 4-digit series airfoils by default have a maximum thickness of 30% of the chord from the leading edge. Therefore with the first 2 digits 00, it indicates that there is no camber and is a symmetrical airfoil.
To find the mean camber line, it can be calculated using: To calculate for the thickness distribution, equation 2. 3 is used. Currently in the market, there are several NACA 4 series airfoil generators. These generators calculate the coordinates of the airfoil shape. However, it does not take into consideration of the load that the airfoil will be taking, meaning the surface area of the wings are not taking into consideration. There is also not much program which enables the user to calculate the whole wing surface area and the lift generated by the wing with different airfoil design. 1. x Thin Airfoil Theory: For this project, the Thin Airfoil Theory will be use to compute the model airplane’s airfoil thickness and wing dimension as the thickness for the model airplane’s wing is not very thick. Thin airfoil theory was devised by German mathematician Max Munk and further refined by British aerodynamicist Hermann Glauert and others in 1920s. It is a simple theory of airfoils that relates the angle of attack to lift. The theory idealizes the flow around an airfoil as a 2 dimensional flow around a thin airfoil.
At low angles of incidence, the boundary layer growth on an airfoil is thin and remains attached to the airfoil. Therefore the airflow is assumed to be inviscid and irrotational. Since the thickness of any lifting airfoil is less than a fifth of its chord length, the effect of the airfoil’s thickness can be neglected and the airfoil will be represented by its mean camber line. A mathematically conceived function in the form of a vortex sheet is then placed along the length of the camber line to simulate the airfoil. This essentially makes it a streamline of the flow.
By applying the circulation theory of lift to this streamline, the aerodynamic properties of the airfoils are obtained. The airfoil is considered as having zero thickness and infinite wingp. This the reason why the theory does not take into consideration the induced drag that arises from the wing tips of an airfoil. Therefore it is only good for approximating an airfoil with medium and large aspect ratio and only up to the stall angle which is usually 10 to 15 degree for typical airplane configuration. Any angle beyond 15 degree, the thin airfoil theory cannot be used to calculate the coefficient of lift.
Thin airfoil theory is important as it provided a theoretical basis for the important properties of the airfoils in 2 dimensional flows: * The centre of pressure lies exactly ? of the chord behind the leading edge on a symmetric airfoil. * The aerodynamic centre lies exactly ? of the chord behind the leading edge for cambered airfoil. * The slope of the lift coefficient versus angle of attack line is 2? units per radian. There are many formulae that states the derivation of the thon airfoil theory, but we don’t require those for our project and so its neglected. xi Flat Plate Airfoil Theory:
Flat plate theory is applied to airfoils as the thickness is also the constituent of drag in the airfoil. The flat plate when it is subjected to airflow the drag minimum as compared to the other shapes with reasonable thickness. Thickness cannot be neglected as it also plays a vital role in the camber surface as it produces lift. The transition point can be pushed back as we reduce the drag in an airfoil by which we can get a good lift curve for higher degrees. In case of boundary layer, the drag in a flat plate will be laminar for a certain period of time and it then become turbulent.
There is no unique valve where the stream gets turbulent, but by keeping the laminar surface clean and free stream, one can delay the renolds number. Figure 2. 10: Velocity profiles for laminar and turbulent boundary layer EXPERIMENTAL PROCEDURE AND ANALYSIS Introduction: These experiments below are done using xflr5 program. NACA 4 digit series airfoils are been used in the analysis. In the final analysis, NACA airfoils ranging from 0 to 9% of camber magnitude, 10 to 70% of camber location and 3% to 30% of thickness airfoils are analysed to get an airfoil of better performance with high to lift to drag ratio.
Maximum lift is at 14 degrees and thus stall occurs. Cl max is 1. 36. It is consistent as Bernoulli’s approach on analysis. Part 2: In this part, the angle of attack is varied from -4 degrees to 18 degrees. Re and M is same as above. The lift curve passes through the origin, so zero lift is at origin (0 degrees). Max Cl is at 1. 36 where the stall angle is at 4 degrees. The maximum glide ratio is 75. 86 and at this point the values of Cl is 0. 8685, value of Cd is 0. 0115 and the value of alpha is 7. 5 degrees. Exercise B: Effect of Reynolds number Airfoil = NACA 0012 Alpha (AOA) = 4 degrees M = 0. 00
Part 1: In this part, we discuss about the effects due to change in Reynolds number in NACA 0012 airfoil. Re number = 100000 to 2. 1 million with an increment of 400000 Figure 3. 6: Pressure profiles plot Part 2: In this part, we discuss about the effects due to change in Reynolds number and Angle of attack in NACA 0012 airfoil. Re number = 100000, 500000, 900000, 2100000, 4000000. AOA (alpha) = -4 to 20 degrees with a step of 0. 5 degrees. Figure 3. 7: Glide ratio and alpha plot Figure 3. 8: Drag coefficient and alpha plot Figure 3. 9: Lift coefficient and alpha plot Figure 3. 10: Lift and Drag polar
Part 2: In this case, the transition point is brought backwards as there is an increase in the boundary layer. It just pushes the stall angle further towards the leading edge. For Re= 100000, drag is increased more at high angle of attack. For high Re number, lift coefficient is more than low Re number and the drag is minimised at high angle of attack in high Re number curves. Exercise C: Effect of Mach number Airfoil = NACA 0012 Re number = 2 million Part 1: In this part, we are discussing about the effects in forces due to change in Mach number. Alpha (AOA) = 4 degrees M = 0. 00, 0. 1, 0. 02, 0. 03 Figure 3. 11: Pressure plot of M=0. 00 Figure 3. 12: Pressure plot of M=0. 01 Figure 3. 13: Pressure plot of M=0. 2 Figure 3. 14: Pressure plot of M=0. 3 Part 2: In this part, we are going to see the difference in the curves for different Mach numbers mentioned in part 1 and also with Angle of attacks from -4 to 30 degrees with a step of 0. 5 degrees.
Part 2: In this part, the angle of attack is also changed with the Mach number. We can see the effect of lift and drag from the values in the table given above in part 2. At the highest AOA the Cl value is increased. As the Mach number increases, the value of Cl max and the stalling angle drops down as it tends to stall soon in high ach number. Exercise D: Effect of Thickness In this exercise, various airfoils of different thickness are used. The values are taken from the program and compared with each other. Re = 2000000, M = 0. 00, AOA(alpha) = -4 to 20 degrees with a step of 0. Airfoils = NACA 0004, NACA 0008, NACA 0012, NACA 0016, NACA 0020 Figure 3. 19: Drag coefficient and Alpha plot Figure 3. 20: Glide ratio and Alpha plot
Symmetrical airfoil is that in which upper and lower surface are same and asymmetrical has various shapes in it. In these two types, the symmetrical airfoil produces less lift-drag ratio than asymmetrical airfoils. Due to the camber in the airfoil, in NACA 1312, NACA 2312 and NACA 3312 has started producing lift at negative angles as other two produces only after 0 degrees. Hence the lift is acquired soon and as angle increases, the drag produced is also less than in airfoils NACA 0012 and NACA 0312. Exercise F: Effect of camber, location
There are minor deflections in the stalling angle as that cannot be accurately calculated. Thus changing the camber location increases lift to some extend as further increase in camber value may cause drag. Exercise G: Finding the best values of a NACA airfoils In this section of analysis, we are using the airfoils of wide range with about 0-9%c camber magnitude, with 10-70%c of camber location and with 3-30% thickness range. We are yet to find the best airfoil with Cl max, largest stalling angle and Cl/Cd(Glide ratio). Re = 2000000, M = 0. 00, AOA(alpha) = -4 to 20 degrees with a step of 0. degrees. Graphs: Figure 3. 30: Lift coefficient and Alpha plot Figure 3. 31: Glide ratio and Alpha plot Figure 3. 32: Glide ratio and Alpha(Re= 200000) Analysis: From the graph 3. 30, we can figure out the maximum Cl value is given by NACA 9318 of about 2. 3. And maximum Cl value when alpha(AOA) is 0 is given by NACA 9718 as per the figure. And hence the airfoil with largest stalling angle also can be seen, as it is NACA 9718. This airfoil though it has less Cl max, it stalls late compared to other airfoils. Its stall angle is going more than 20 degrees.
Cite this Page
Aerofoil Design Xflr5. (2018, Jul 01). Retrieved from https://phdessay.com/aerofoil-design-xflr5/
Run a free check or have your essay done for you
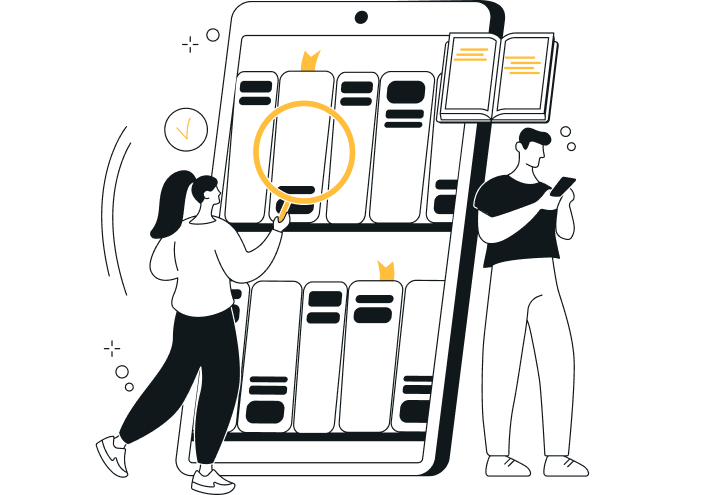