Diversities in reproductive modes and strategies amongst various reptile species
Abstract
In this paper we look at reptile reproduction and the how the different factors and processes involved vary in different species. We look at:
Reproductive cycles
Order custom essay Diversities in reproductive modes and strategies amongst various reptile species with free plagiarism report
Spermatogenesis and oogenesis
Copulation and Fertilization
Egg shells
Ovoviviparity
Viviparity
Parthenogenesis
Parental body condition
Reproductive behaviour
Parental care
Reproductive effort as a life history trait
Energy expenditure per progeny
Offspring size
Sex determination
Environmental factors that affect reproduction
Introduction
Reproduction is a hugely important aspect of conservation, and with 106 species of reptile being classed as “Critically Endangered” on the IUCN Red List of Threatened Species [1], every bit of knowledge could be useful for preventing a species becoming extinct.
Reproduction is highly varied amongst the different species of reptile, and the three main reproductive modes are oviparity, ovoviviparity and viviparity.
All the different modes and methods stem from the unique evolutionary challenges that each different species has encountered, having to adapt its reproductive processes to avoid extinction.
Even amongst species that have similar reproductive modes, there are distinct variations in anatomy, physiology and behaviour, ranging from the obscure to the ridiculous, from self-fertilising, all-female lizard species, to “living fossils” that mate by cloacal apposition, due to the male’s lack of a penis.
Other, less unusual, variations occur in reproductive cycles, clutch size, mating and parental behaviour.
Reproductive cycles
For reproduction to be successful, both the males and females need to be behaviourally and physiologically capable and ready to mate and reproduce.
Reptiles tend to exhibit 3 different types of reproductive cycle :
Associated cycle
Dissociated cycle
Continuous cycle
Associated cycles are common and are frequently found in species that live in temperate zones and seasonally tropical environments, as these regions have relatively long periods suitable for reproduction . In species with an associated cycle, oogenesis and spermatogenesis are initiated simultaneously. High levels of sex hormones present tend to be associated with gamete maturation, mating and fertilization .
An example is the green anole, Anolis carolinensis, from southeastern United States. In these, male and female gonads develop simultaneously in the spring, mating and egg production occur in the summer, and both male and female gonads regress in the autumn .
Dissociated cycles occur in viviparous species that live in areas where there is a short breeding season, and the best time for mating may not necessarily be the best time for production of young .
An example is the red-sided garter snakes, Thamnophis sirtalis parietalis. These mate after emerging from hibernation in the spring, but the gonads are not fully functional and sex steroids are at a low level. The sperm that was used in the mating was produced the previous summer and was stored in the male’s vas deferens. The female’s eggs mature during the summer, and become fertilized by the stored sperm from the spring mating [4]. This allows mating to occur at denning, when the males and females are together, and allows the snake to use the rest of its active time to mature and gestate [4, 6].
Continuous reproductive cycles allow the animal to reproduce at any point throughout the breeding season [5]. They commonly occur in species that live in tropical habitats . An example is the Amazonian Basin teiid, Neusticurus ecpleopus, where males have “mature spermatozoa in the right testis and epididymis during every month of the year” and so can reproduce all year round.
Spermatogenesis and oogenesis
Spermatogenesis in reptiles is similar to that of most other vertebrates . Spermatogenesis occurs in the reptile’s testes; however, it has been noted that reptilian germ cells are not organised into “consistent cellular associations” , and spermatids can be grouped with others from different mitotic and meiotic generations.
In the six-lined racerunner, Aspidoscelis sexlineatus, spermatids from the male’s vas deferens have bent midpieces, whereas spermatids from the female’s oviducts have straight midpieces. This indicates that the final stage of maturation of the spermatids occurs in the female’s oviduct after copulation .
Spermatogenesis in temperate species is limited to summer, when it is warmer and food more abundant. Therefore energy is more readily available for spermatogenesis, which is metabolically demanding . In contrast, spermatogenesis is a continuous process in tropical reptiles .
Reptilian oogenesis occurs in the ovaries, which are the site of yolk deposition [12]. Vitellogenesis occurs in the maternal liver, and yolk consists of glycogen, lipoproteins and phosphorylated proteins [6].
The ova is released from the ovary surface and enters the oviduct at the ostium, the oviduct’s anterior opening [6]. Albumin deposition occurs in the oviduct and at a location which is dependent on species. Crocodilians and chelonians have albumin deposited on the ovum in the tube section of the oviduct, whereas in squamate reptiles it is deposited in the posterior part of the infundibulum .
Copulation and Fertilization
All reptile species have internal fertilization, but can accomplish this in different ways, depending on anatomy, environment and other factors .
Sphenodontids, such as tuatara from New Zealand, first perform a conspicuous courtship, followed by copulation. They copulate by a process of cloacal apposition, as the males lack a distinctive intromittent organ ].
Some species, such as lacertid lizards and all snakes, have a fully paired reproductive system, including intromittent organs, known as the hemipenes. They are independent and set on either side of the midline .
The hemipenes develop as paired evaginations from the back of the cloaca and, when flaccid, they are inverted, but in order to mate, they fill with blood and evert . It is possible to identify species of a colubrid snake from the size, shape and weight of the hemipenes .
The common garter snake, Thamnophis sirtalis, favours the right-hand hemipenis, much like humans being lefthanded or righthanded. The right hemipenis is wider and longer than the left .
Male chelonians have a penis with a single “midline groove that lies between two longitudinal ridges”. These ridges allow the penis to engorge with blood when mating. The penis is not associated with the urinary system, and used purely for reproduction .
After the male reptile has ejaculated, the females of many reptilian species can store the sperm if the reproductive cycle of the male and female do not coincide .
In snakes, mating can occur several months in advance and the females can store the sperm in the infundibulum; an example is the South American rattlesnake, Crotalus durissus. Female lizards typically store the sperm in the utero-vaginal transition of the oviduct, as seen in the little brown skink, Scincella lateralis .
Fertilization occurs when the ova enter the oviduct and meet the sperm .
Egg shells
Many species of reptile are oviparous and lay eggs, which can be divided into 3 main groups:
Flexible-shelled eggs with little or no calcareous layer
Flexible-shelled eggs with a thick, well-developed calcareous layer
Rigid-shelled eggs with a well-developed calcareous layer
The structure and thickness of the shell membrane and the mineral layer varies between species .
Most squamate reptiles produce eggs that have a very flexible shell ; some even lack the calcareous mineral layer. The desert iguana, Dipsosaurus dorsalis, has only a fibrous shell membrane . Although the green iguana, Iguana iguana, also is missing the calcareous layer, it still retains “isolated crystals of calcareous material among the fibres of the shell membrane” .
Some squamate reptile eggs may retain the calcareous layer, as in A. carolinensis, the eggs of which have a thin calcareous crust , organised into an irregular array of plaques, “with underlying fibrous layers of the membrane being visible between the plaques” .
Turtles, including chelonids, chelydrids and most emydids, produce eggs that have a flexible shell, with a well-defined calcareous layer roughly the same thickness as the shell membrane . In sea turtles, the calcareous material forms a loosely organized open matrix, whereas in emydids and chelydrids, the eggshell has calcareous material that is much more highly structured .
Rigid eggshells are laid by a number of different species, including:
Crocodilians
Some chelonians
Many species of gecko
Rigid eggs have a thick, well-developed calcareous layer forming the majority of the shell .
For the embryo to survive inside the egg, the shell needs to be partially permeable allowing gaseous exchange. Early in development, the first gaseous exchange occurs in the yolk sac, and later in the chorioallantois . The majority of gaseous exchange occurs after oviposition, in the air in the nest chamber.
The shells can be partially permeable to water, and different species manage this in various ways. In some, the egg will contain all the water required for embryo development at oviposition, as seen in:
Crocodilians
Tuataras
Some turtle species
Most squamate reptile eggs have a low water content at oviposition, and need to absorb water from the environment for successful development of the embryo.
Ovoviviparity
Ovoviviparity occurs when the embryo develops inside an egg, but the egg is retained inside the mother until ready to hatch . Despite the embryo being retained, there is no well-developed placenta and the remains of a shell can be found during early embryonic development .
In the spiny lizard, Sceloporus jarrovi, ovulation and fertilization occur between late November and early December, but embryonic development is suspended for 4 months in the blastoderm stage. At this point, the embryo is encased in a shell and nutrients are supplied by the egg yolk. After development continues, placental formation occurs, and the yolk plays a lesser role. “Delayed development ensures an adequate food supply for the young at parturition and eliminates embryonic development during winter when adult food intake is limited” .
The viviparous lizard, Lacerta vivipara, has some populations that are ovoviviparous, and these have “substantial hormone leakage between mother and offspring and between fetuses”, and so maternal hormone levels and stress are likely to have a significant impact on the embryonic development. Stress has been shown to influence thermal regulation , which can affect sexual determination of the offspring .
Viviparity
A viviparous reptile will retain the embryos in the oviduct until the neonate is fully formed and is born. The majority of reptiles are oviparous, but there are around 100 species of lizard and snake that are viviparous, including:
Alligator lizards, Elgaria coerulea
Boa constrictor, Boa constrictor
Many geckos
Many species of skink
Turtle-headed sea snakes, Emydocephalus ijimae
As there is a large variety of viviparous reptiles, it is not surprising that they exhibit “all grades of embryonic nutrition” , ranging from an almost total dependence on the stores of egg yolk, to a total reliance on maternal nutrient transfer.
The South American skink, Mabuya heathi, is viviparous and produces newly ovulated eggs that are around 1mm in diameter. The embryo obtains more than 99% of its nutrition from placental transfer from the mother. Pregnancy lasts 8-12 months and the majority of the placental nutrient provision occurs in the last 3 months of gestation.
There is no agreed theory why only squamate reptiles show viviparity and none in chelonians or crocodilians. Recent theories suggest that it evolved in colder climates, as the embryo can benefit from maternal thermoregulatory behaviour, such as basking, allowing the embryo to develop faster .
This does not mean that viviparity is restricted to cooler regions, and a number of viviparous species of the genus Sceloporus, exist in both warm and cool regions of varying altitude in the south-west of America and in Mexico .
Parthenogenesis
The majority of reptile species reproduce sexually, but a small number of species are able to reproduce parthenogenetically. Asexual reptiles often have three common features: they originate from the hybridization of two species, reproduction is from clonal inheritance and all are female .
Unisexual reptiles were first noted in 1935, when it was noted that there had never been any male fox gecko, Hemidactylus garnoti, observed . Parthenogenesis has been reported in over 30 species of squamate reptile, mostly xantusiids, gekkonids, lacertids and teiids . There is only one species of snake that is parthenogenetic, the Brahminy blind snake, Ramphotyphlops braminus.
Parthenogenesis reduces the costs of reproduction, as there is no energy invested in the males, and each individual female can reproduce independently. It has the disadvantage that the progeny will not easily adapt to changes in environment.
The parthenogenic females produce diploid eggs from unreduced gametes, so all offspring are genetically identical to the parent .
Unisexual species occur as a result of hybridization, and “each unisexual vertebrate species combines at its inception much of the genetic diversity of two different sexual species” . There are no intermediate forms, backcross genome combinations or hybrid zones that involve the parental taxa .
Parthenogenetic species do not need to reproduce sexually, but sexual behaviour does still occur. Some parthenogenetic species of whiptail lizard, Cnemidophorus, have been seen to mount others in pseudocopulation . This behaviour is almost identical to that of Cnemidophori lizards that are not parthenogenetic . This occurs under crowded conditions and probably is not related to reproduction .
Parental body condition
Reproduction is energy-expensive for most species, but the degree varies with species and gender .
“Capital breeders” rely on energy that has been stored as fats or proteins, rather than relying on energy intake . This suggests that there is a threshold amount of energy that is required to be stored before the animal is capable of reproduction . In reptiles, it is often associated with ambush predators, such as the European asp, Vipera aspis.
Only females have an energy threshold that is required prior to breeding, and female V. aspis will not attempt to reproduce until “they exceed a certain minimal level of body reserves for vitellogenesis” . In males, there is no energy-dependent threshold, and in V. aspis, there is no noticeable difference in body condition between reproductive and non-reproductive males . However, it has been noted that there is a decrease in feeding behaviour in male vipers during the breeding season, as males devote more time and energy to finding a mate.
Captive breeding ofSaint Croix’s anole, Anolis acutus, in the Caribbean, has shown that an increase in food intake has no effect on the reproductive effort of females, but it did cause an increase for males. Reproductive effort is calculated as 100 x gonad weight / lean body weight .
Another reptile where body size and condition show no effect on reproduction and mean egg clutch size is Rodrigues’ lava lizard, Eurolophosaurus nanuzae. In this species, female reproduction is instead influenced by photoperiod, long-term precipitation and temperature.
“Income breeders” do not store energy for reproduction; instead reproduction is fuelled by feeding . It is usually a linear relationship, where an increase in the energy available results in an increase in reproductive output .
The Oriental garden lizard, Calotes versicolor, can be found in regions where there is an abundance of food. It maintains a store of abdominal body fat, which is used to provide energy for vitellogenesis for the first clutch of the season. After this, they switch to being income breeders and rely on constant daily feeding to produce enough energy for any consequent clutches of eggs.
Reproductive behaviour
Every reptile species has its own specific reproductive behaviour associated with mating, nesting and caring for the young.
In the male T. sirtalis parietalis, the choice of female is influenced by the characteristics and attributes of the female. In garter snakes, it has been shown that larger, heavier females are more desirable to the males and attract more courtship than smaller, thinner snakes].
It can be estimated that the intensity of the mating behaviour in most male reptiles is influenced by three factors:
Phenotypic aspects that influence ability to compete
Potential fitness benefits from mating
Intensity of competition for mating
Male T. sirtalis parietalis display most courtship behaviour when alone with a female, and if there are many males all competing for the same female then they show a significant reduction in courtship behaviour .
In other reptile species, the male can have displays that could consist of coloured skin patches and loud calling or unusual activities, such as in the “push-up displays” of the male side-blotched lizard, Uta stansburiana . The disadvantage of this display is a reduced endurance in the leg muscle, which may be needed to escape a predator.
Male ornate crevice-dragons, Ctenophorus ornatus, have brightly coloured patches of skin on their chests. These can be used to predict the size of a male’s territory and how many females live in that territory .
Ritual combat allows males to compete for dominance prior to mating, and the winner often gets the female. These battles consist of entwining with and pushing the opponent to the ground to subdue him. This is seen in a number of species, including:
Australian blacksnakes, Pseudechis porphyriacus
Western rattlesnake, Crotalus viridis
Many colubrids, including Chironius bicarinatus
Tuatara, Sphenodon punctatus
Snouted cobras will refuse to mate unless they have performed a combat ritual with another male .
It is rare that the female has a choice in partners, and in C. ornatus, the females show no preference amongst different males, despite the varying intensity and colour of the male’s display patches . This could mean that the colours are for male dominance competitions rather than luring a mate. Female choice has only been conclusively proven in a few species, including Lacerta agilis and several Anolis lizards .
In leopard geckos, Eublepharis macularius, the sexual and agonistic behaviour can be affected by the gonadal sex and the incubation temperature, as they both affect sex steroid hormone release . Female leopard geckos that were incubated at an intermediate temperature were less “attractive” to the male geckos than females that were incubated at warmer temperatures .
Parental care
The benefit of parental care over a clutch of eggs could be to increase the survival rate of the eggs. The parental care may be in the form of defending the nest site, egg brooding by muscular thermogenesis or egg attendance.
Different reptile species display differing amounts of parental care. The majority do not exhibit any parental care, such as turtles. Those exhibiting parental care include:
Crocodilians
Long-tailed skinks, Mabuya longicaudata
Skinks of the genus Eumeces
Pythons
Asian forest tortoise, Manouria emys
Female crocodilians give large amounts of parental care to both the eggs and the young after hatching. The females will remain close to the nest and defend it from potential predators. The male is not present when the eggs are laid but there may still be some male role in nest protection.
Young Nile crocodiles, Crocodylus niloticus, vocalise while still in the egg and continue to do so after hatching . These calls lead the mother to the nest, who then opens it, help the young escape from the shell and carries the hatched young to water . After hatching, the young may also give distress calls which would stimulate defensive behaviour by the adults . It is possible that the young could be calling to each other, to synchronise hatching and ensure survival from predators .
Snakes exhibiting parental care usually do so in the form of egg attendance and defensive behaviour, usually in species that are either large or venomous, as these can easily defend the nests.
Pythons participate in egg brooding which keeps the eggs warm by muscular thermogenesis, but in Children’s pythons, Antaresia childreni, excessive brooding can smother the eggs, causing hypoxia due to the partially permeable shells. This can give “smaller, slower and weaker” offspring than the offspring of pythons that do not egg-brood.
Reproductive effort as a life history trait
The amount of effort expended in reproduction can affect the survival of the animal or can affect future reproduction, and so a trade-off is required In squamate reptiles, survival costs often result from the “risk of death associated with a reproductive event” , an example being that a gravid female has a reduced mobility and is easily predated upon.
Reproduction requires increased energy and so the animal will require a higher food intake, both before copulation and during pregnancy. The foraging or hunting may make them more at risk from predators .
Reproductive costs arise because the animal devotes energy to its current reproductive bout. This energy cannot be used for growth of the animal and will not give a higher fecundity during a later reproductive bout .
In squamate reptiles, survival costs are considered more important than reproductive costs, and the trade-off between present and future fecundities may not be significant for many species
The counter arguments to this are that “variation in the form of trade-offs relating offspring size and survival substantially affect relationships among clutch size, relative clutch mass, and lifetime reproductive success”, and that the way in which adult mortality is simulated in the mathematical model can significantly affect the conclusions about the potential fecundity trade-offs .
The environment may affect the reproductive effort, destabilising the trade-offs. An example of this would be an individual that is in a poor condition, which could result in it getting a poor territory and suffering increased mortality or reduced fecundity as a result .
Both survival and reproduction costs can be seen in the tree lizard, Urosaurus ornatus in New Mexico, which lays two clutches of eggs during the summer. It has been shown that reducing the eggs in the first clutch, by surgically reducing the number of follicles, resulted in females that had a higher survival rates, and larger second clutches .
Many species with short life ps tend to have multiple clutches each year and have a high clutch mass to female mass ratio. However, many species with long life ps tend to be more restrained with their reproductive activities .
Energy expenditure per progeny
The energy invested in an egg by the mother has an effect on the size and health of the neonate in oviparous reptiles . Females can produce either large clutches of many small eggs or small clutches of large eggs .
Female veiled chameleon, Chamaeleo calyptratus produce clutches of 20-40 eggs, each weighing 1.5g . Female Fiji banded iguana, Brachylophus fasciatus are the same size but produce clutches of 3-4 eggs, each weighing 10g .
In some species, the eggs are smaller than optimum due to the structural constraint of the size of the female’s pelvis. The eggs of the painted turtle, Chrysemys picta are proportional to the width of the pelvis opening, so larger females lay larger eggs . By contrast, all lizards in the genus Anolis produce only one egg in each clutch, and this egg is sized so that it is the largest that can possibly fit through the pelvis .
In several species of lizard, the clutch size is fixed and “the female is not able to divide the energy invested during the single reproductive bout into more offspring”. These species include:
Anolis
Gekkonidae
Dibamidae
Some skinks
The size of the egg generally increases with the size of the species, but it is not directly proportional. Larger species have smaller offspring relative to their adult body size than do small species .
Some species that have variable clutch sizes include:
Crocodilians
Tuataras
Most turtles
Many squamate retiles
The total number of offspring in a clutch is ultimately dependent on the number of follicles produced, the proportion of follicles that become vitellogenic and the incidence of atresia of vitellogenic follicles prior to ovulation .
It has been noted that other factors can affect the size of the clutch produced. An increased environmental temperature earlier in the year has been shown to lead to earlier breeding, as well as giving an increased clutch size, possibly due to “changes in the reproductive cycle and energy expenditure” .
Offspring size
All offspring produced by oviparous reptiles have characteristics that are “highly dependent upon the physical conditions that the embryo experiences prior to hatching” , such as its time of hatching, performance abilities, size, shape, behaviour and thermoregulatory preferences.
There is an inverse relationship between offspring size and clutch size, but this can be influenced by maternal body size .
Studies into the spiny lizard, Sceloporus virgatus, have shown that there is no correlation between egg mass and maternal body mass . This is different to most turtle species, where the maximum possible egg size is limited by the diameter of the pelvic opening .
Snakes are not constrained by the pelvic girdle, and so there is a positive correlation between the width of the egg laid and the female’s mass.
Eggs in the same clutch tend to give uniform-sized offspring. There are rarely overly large or small offspring, possibly because eggs have been exposed to similar hydric and thermal conditions throughout embryogenesis, and so have all developed in the same way .
The phenotype of the reptile hatching out of the egg can be significantly influenced by the “thermal regimes selected by the gravid female immediately prior to laying”, as well as food availability to the gravid female.
If the female lays large numbers of eggs, then the energetic and nutritional variance within the clutch is low for reptiles, compared with birds .
Sex determination
In reptile species, gender can be determined either by genetics or temperature In temperature-dependent sex determination of species that lay eggs, the temperature of the eggs is controlled by the nest temperature, as with egg-brooding pythons . In viviparous species, the temperature is controlled by basking.
The thermoregulatory history of viviparous reptiles can affect a number of characteristics of the offspring, including:
Size
Phenotype
Survival
Locomotor performance
Behaviour
At temperatures that produce females, the enzyme aromatase is induced and converts testosterone to oestradiol, which binds to oestrogen receptors, triggering feminisation. At temperatures that produce males, the enzyme 5?-reductase is induced and converts testosterone to dihydrotestosterone, which binds to androgen receptors, triggering differentiation of testes .
In snapping turtles, eggs developing at “female producing temperatures” have a hormonal environment in which oestradiol concentrations decline at a slower rate than those at “mixed sex temperatures” or “male producing temperatures” .
There are three patterns of temperature-dependent sex determination:
Males at cool temperatures, females at warm temperatures
Females at cool temperatures, males at warm temperatures
Females at coolest and warmest temperatures, males at intermediate temperatures
The range of temperatures that can give both sexes is typically only 1°C and varies for each species. The temperature that gives 50% of each sex is known as the “pivotal temperature” .
All crocodilian species exhibit temperature-dependent sex determination . In the black caiman, Caiman latirostris, incubating the eggs at 29-31°C gives female offspring, and incubating at 33°C gives male offspring. At 34.5°C, both male and female are hatched
In the American alligator, Alligator mississippiensis, there are three types of nesting site, which all have differing temperatures :
Levee? 34°C
Wet marsh? 30°C
Dry marsh ? 34°C on top and 30°C at peripheries
Alligators nesting in wet marshes produce nearly 100% female offspring, and females “tend to seek a temperature environment that is as close as possible to that of their own incubation” . This gives a skewed sex ratio of nearly 10 female alligators to 1 male.
Temperature-dependent sex determination was originally thought to occur exclusively in species that lack sex chromosomes, but it has been shown to co-exist with genetic-dependent sex determination in some species, including geckos and some skinks
Environmental factors that affect reproduction
It has been shown that environmental and nest temperatures can affect the gender of the hatchlings , and variations in temperature can also affect factors such as foraging, breeding seasons and hibernation .
Climate warming in China has been shown to shift the oviposition dates of the Chinese alligator, Alligator sinensis, to earlier in the year, as well as to increase the mean clutch size .
Ecological events in the area inhabited by a reptile can also affect the reproduction, and human land-management can be a major factor. The desert horned lizard, Phrynosoma platyrhinos, will prefer to inhabit areas where cattle grazing has occurred, presumably due to an abundance of prey species. This would mean that there would be increased reproductive rates.
In southern Texas, burning scrubland in the winter is commonplace, and can have an effect on the reptile populations. “Winter burning provides an increase in food resources and leads to increased survival of Texas horned lizards, Phrynosoma cornutum, in the second growing season after fire” .
In other cases, habitat loss may have an adverse effect on the reproduction of reptiles. In Argentina, deforestation has been shown to have an effect on the Argentine boa constrictor, Boa constrictor occidentalis, particularly causing a decrease in body condition, clutch size and testicular volume .
Conclusions
Species variations in reptile reproduction may be quite large, with some species able to reproduce at any time throughout the year and others having dissociative reproductive cycles where the female can “store” the male’s sperm . Other aspects are typically less varied; spermatogenesis and oogenesis are similar in most species .
Anatomical species differences can be seen in the structure of the male reproductive organs. Some, such as tuatara lack a penis at all , whereas snakes have two hemipenes . In testudines the penis is used solely for reproduction and is not even connected to the urinary tract .
Reptilian life-history traits can vary between species and include clutch size, offspring size and mode of parity.
Some reptile species are oviparous and lay eggs, such as the pit viper, Trimeresurus flavoviridis , some oviparous species are even parthenogenetic and all-female. Others are ovoviviparous, like S. jarrovi and others are viviparous and produce live young, such as M. heathi .
Reproductive behaviour also varies between species and can help the reptile select a suitable mate and parental care can help ensure the survival of the offspring, even if it is detrimental to the health of the parent .
There is a distinct trade-off between reproductive effort and survival of the offspring and the parent, and different species cope with this in different ways. Some produce large clutches and have only a small proportion of these survive to adulthood whereas others have smaller clutches with a higher survival ratio .
References
1. IUCN. IUCN Red List of Threatened Species. Version 2010.4. 2011 10th April 2011]; Available from: http://www.iucnredlist.org.
2. Jones, R.E. and D.O. Norris, Hormones and reproduction in fishes, amphibians, and reptiles / edited by David O. Norris and Richard E. Jones, 1987, New York : Plenum Press, c1987.
3. Jenssen, T.A., M.B. Lovern, and J.D. Congdon, Field-Testing the Protandry-Based Mating System for the Lizard, Anolis carolinesis: Does the Model Organism Have the Right ModelBehavioral Ecology and Sociobiology, 2001. 50(2): p. 162-172.
4. Crews, D. and R.G. William, The Ecological Physiology of a Garter Snake. (cover story). Scientific American, 1982. 247(5): p. 158-168.
5. Sherbrooke, W.C., Reproductive Cycle of a Tropical Teiid Lizard, Neusticurus ecpleopus Cope, in Peru. Biotropica, 1975. 7(3): p. 194-207.
6. Harvey Pough, A., Cadle, Crump, Savitzky, Wells, Herpetology2004.
7. Rua, M. and P. Galan, Reproductive characteristics of a lowland population of an alpine lizard: Lacerta monticola (Squamata, Lacertidae) in north-west Spain. Animal Biology, 2003. 53(4): p. 347-366.
8. Gribbins, K.M., et al., Continuous spermatogenesis and the germ cell development strategy within the testis of the Jamaican Gray Anole, Anolis lineatopus. Theriogenology, 2009. 72(4): p. 484-492.
9. Newton, W.D. and S.E. Trauth, Ultrastructure of the Spermatozoon of the Lizard Cnemidophorus sexlineatus (Sauria: Teiidae). Herpetologica, 1992. 48(3): p. 330-343.
10. Olsson, M., T. Madsen, and R. Shine, Is sperm really so cheapCosts of reproduction in male adders, Vipera berus.
11. Hernandez-Gallegos, O., et al., Continuous Spermatogenesis in the Lizard Sceloporus bicanthalis (Sauria: Phrynosomatidae) from High Elevation Habitat of Central Mexico. Herpetologica, 2002. 58(4): p. 415-421.
12. Hei, N., et al., Fine structural observation on the oogenesis and vitellogenesis of the Chinese soft-shelled turtle ( Pelodiseus sinensis). Zygote (Cambridge, England). 18(2): p. 109-120.
13. Guillette, L.J., S.L. Fox, and B.D. Palmer, Oviductal Morphology and Egg Shelling in the Oviparous Lizards Crotaphytus-Collaris and Eumeces-Obsoletus.
14. Moore, J.A., et al., Seasonal monogamy and multiple paternity in a wild population of a territorial reptile (tuatara). Biological Journal of the Linnean Society, 2009. 98(1): p. 161-170.
15. Arnold, E.N., The hemipenis of lacertid lizards (Reptilia: Lacertidae): structure, variation and systematic implications. Journal of Natural History, 1986. 20(5): p. 1221.
16.Shine, R., et al., Are snakes right-handed Asymmetry in hemipenis size and usage in gartersnakes (Thamnophis sirtalis). Behavioral Ecology, 2000. 11(4): p. 411-415.
17. Branch, W.R., Hemipenial Morphology of African Snakes: A Taxonomic Review. Part 1. Scolecophidia and Boidae. Journal of Herpetology, 1986. 20(3): p. 285-299.
18. Dowling, H.G., Hemipenes and Other Characters in Colubrid Classification. Herpetologica, 1967. 23(2): p. 138-142.
19. Rivera, S., Health Assessment of the Reptilian Reproductive Tract. Journal of Exotic Pet Medicine, 2008. 17(4): p. 259-266.
20. Yamanouye, N., et al., Reproductive cycle of the Neotropical Crotalus durissus terrificus: II. Establishment and maintenance of the uterine muscular twisting, a strategy for long-term sperm storage. General & Comparative Endocrinology, 2004. 139(2): p. 151-157.
21. Sever, D.M. and W.A. Hopkins, Oviductal sperm storage in the ground skink Scincella laterale holbrook (Reptilia : Scincidae).
22. Packard, M.J., G.C. Packard, and T.J. Boardman, Structure of Eggshells and Water Relations of Reptilian Eggs. Herpetologica, 1982. 38(1): p. 136-155.
23. Rand, A.S., Desiccation Rates in Crocodile and Iguana Eggs. Herpetologica, 1968. 24(2): p. 178-180.
24. Andrews, R. and A.S. Rand, Reproductive Effort in Anoline Lizards. Ecology, 1974. 55(6): p. 1317-1327.
25. Packard, G.C., et al., Influence of Moisture, Temperature, and Substrate on Snapping Turtle Eggs and Embryos. Ecology, 1987. 68(4): p. 983-993.
26. Packard, G.C., et al., Changes in Mass of Softshell Turtle (Trionyx spiniferus) Eggs Incubated on Substrates Differing in Water Potential. Herpetologica, 1979. 35(1): p. 78-86.
27. Phillott, A.D. and C.J. Parmenter, The ultrastructure of sea turtle eggshell does not contribute to interspecies variation in fungal invasion of the egg. Canadian Journal of Zoology, 2006. 84(9): p. 1339-1344.
28. Grahame, J.W.W. and C.-P. Harvey, Effects of Incubation Temperature on Crocodiles and the Evolution of Reptilian Oviparity. American Zoologist, 1989. 29(3): p. 953-971.
29. Deeming, D.C. and T.R. Whitfield, Effect of shell type on the composition of chelonian eggs.
30.Packard, G.C., Water Relations of Chelonian Eggs and Embryos: Is Wetter BetterAmerican Zoologist, 1999. 39(2): p. 289-303.
31.Kratochvil and D. Frynta, Body-size effect on egg size in eublepharid geckos (Squamata: Eublepharidae), lizards with invariant clutch size: negative allometry for egg size in ectotherms is not universal. Biological Journal of the Linnean Society, 2006. 88(4): p. 527-532.
32. Thompson, M.B. and K.J. Russell, Growth and energetics of embryos of the gecko, Phyllodactylus marmoratus, a species with hard-shelled eggs.
33. Sexton, O.J., et al., Eggshell composition of squamate reptiles: Relationship between eggshell permeability and amino acid distribution.
34. Kratochvil, L. and D. Frynta, Egg shape and size allometry in geckos (Squamata: Gekkota), lizards with contrasting eggshell structure: why lay spherical eggsJournal of Zoological Systematics & Evolutionary Research, 2006. 44(3): p. 217-222.
35. Nicola, J.N., et al., Egg mass determines hatchling size, and incubation temperature influences post-hatching growth, of tuatara Sphenodon punctatus. Journal of Zoology, 2004. 263(1): p. 77-87.
36. Uller, T. and M. Olsson, Direct Exposure to Corticosterone During Embryonic Development Influences Behaviour in an Ovoviviparous Lizard. Ethology, 2006. 112(4): p. 390-397.
37. Goldberg, S.R., Reproductive Cycle of the Ovoviviparous Iguanid Lizard Sceloporus jarrovi Cope. Herpetologica, 1971. 27(2): p. 123-131.
38. Cree, A., et al., Protecting embryos from stress: corticosterone effects and the corticosterone response to capture and confinement during pregnancy in a live-bearing lizard (Hoplodactylus maculatus). General And Comparative Endocrinology, 2003. 134(3): p. 316-329.
39. Andrews, R.M., Incubation Temperature and Sex Ratio of the Veiled Chameleon (Chamaeleo calyptratus). Journal of Herpetology, 2005. 39(3): p. 515-518.
40. Robert, K.A. and M.B. Thompson, Viviparity and temperature-dependent sex determination. Sexual Development: Genetics, Molecular Biology, Evolution, Endocrinology, Embryology, And Pathology Of Sex Determination And Differentiation. 4(1-2): p. 119-128.
41. Shine, R., Does Viviparity Evolve in Cold Climate Reptiles Because Pregnant Females Maintain Stable (Not High) Body TemperaturesEvolution, 2004. 58(8): p. 1809-1818.
42.Vitt, L.J. and W.E. Cooper, Jr., Maternal Care in Skinks (Eumeces). Journal of Herpetology, 1989. 23(1): p. 29-34.
43. Wen-san, H. and W. Hurng-Yi, Predation Risks and Anti-Predation Parental Care Behavior: An Experimental Study in a Tropical Skink. Ethology, 2009. 115(3): p. 273-279.
44. Masunaga, G., et al., Reproductive biology of the viviparous sea snake Emydocephalus ijimae (Reptilia : Elapidae : Hydrophiinae) under a seasonal environment in the northern hemisphere.
45. Blackburn, D.G. and L.J. Vitt, Specializations of the chorioallantoic placenta in the Brazilian scincid lizard, Mabuya heathi: A new placental morphotype for reptiles.
46. Zhang, D.J., et al., Effect of gestation temperature on sexual and morphological phenotypes of offspring in a viviparous lizard, Eremias multiocellata.
47. Mendez-de la Cruz, F.R., M.V.n.-S. Cruz, and R.M. Andrews, Evolution of Viviparity in the Lizard Genus Sceloporus. Herpetologica, 1998. 54(4): p. 521-532.
48. Maslin, T.P., Parthenogenesis in Reptiles. American Zoologist, 1971. 11(2): p. 361-380.
49. The fauna of British India, including Ceylon and Burma. [Vertebrata]: Reptilia and amphibia by M.A. Smith.
50. Neaves, W.B. and P. Baumann, Unisexual reproduction among vertebrates. Trends in Genetics. 27(3): p. 81-88.
51.Kamosawa, M. and H. Ota, Reproductive Biology of the Brahminy Blind Snake (Ramphotyphlops braminus) from the Ryukyu Archipelago, Japan. Journal of Herpetology, 1996. 30(1): p. 9-14.
52.Cole, C.J. and C.R. Townsend, Sexual behaviour in unisexual lizards. Animal Behaviour, 1983. 31(3): p. 724-728.
53. Leuck, B.E., Comparative Social Behavior of Bisexual and Unisexual Whiptail Lizards (Cnemidophorus). Journal of Herpetology, 1985. 19(4): p. 492-506.
54. Aubret, F., et al., Fat Is Sexy for Females but Not Males: The Influence of Body Reserves on Reproduction in Snakes (Vipera aspis). Hormones & Behavior, 2002. 42(2): p. 135.
55. Bonnet, X., Capital versus income breeding: an ectothermic perspective. Oikos, 1998. 83(2): p. 333-342.
56. Jonsson, K.I., Capital and income breeding as alternative tactics of resource use in reproduction. Oikos, 1997. 78(1): p. 57-66.
57. Naulleau, G. and X. Bonnet, Body Condition Threshold for Breeding in a Viviparous Snake. Oecologia, 1996. 107(3): p. 301-306.
58. Bonnet, X. and G. Naulleau, Are Body Reserves Important for Reproduction in Male Dark Green Snakes (Colubridae: Coluber viridiflavus)Herpetologica, 1996. 52(2): p. 137-146.
59. Rose, B., Food Intake and Reproduction in Anolis acutus. Copeia, 1982. 1982(2): p. 322-330.
60. Assis, V.c.B., et al., Reproduction and Fat Body Cycle of Eurolophosaurus nanuzae (Sauria; Tropiduridae) from a Seasonal Montane Habitat of Southeastern Brazil. Journal of Herpetology, 2003. 37(4): p. 687-694.
61. Shanbhag, B.A., Reproductive strategies in the lizard, Calotes versicolor.
62. Langkilde, T., R. Shine, and R.T. Mason, The Opportunistic Serpent: Male Garter Snakes Adjust Courtship Tactics to Mating Opportunities. Behaviour, 2003. 140(11/12): p. 1509-1526.
63. Aleksiuk, M. and P.T. Gregory, Regulation of Seasonal Mating Behavior in Thamnophis sirtalis parietalis. Copeia, 1974. 1974(3): p. 681-689.
64. Shine, R., et al., Pick on someone your own size: ontogenetic shifts in mate choice by male garter snakes result in size-assortative mating.
65. Shine, R. and P. Harlow, The transvestite serpent: Why do male garter snakes court (some) other malesAnimal Behaviour, 2000. 59(2): p. 349.
66.Shine, R., D. O’Connor, and R.T. Mason, Female mimicry in garter snakes: behavioural tactics of “she-males” and the males that court them.
67. Sullivan, B.K. and M.A. Kwiatkowski, Courtship displays in anurans and lizards: theoretical and empirical contributions to our understanding of costs and selection on males due to female choice. Functional Ecology, 2007. 21(4): p. 666-675.
68. Brandt, Y., Lizard threat display handicaps endurance. Proceedings of the Royal Society B: Biological Sciences, 2003. 270(1519): p. 1061-1068.
69. Shine, R., et al., Mating and Male Combat in Australian Blacksnakes, Pseudechis porphyriacus. Journal of Herpetology, 1981. 15(1): p. 101-107.
70. Aldridge, R.D., Male Reproductive Anatomy and Seasonal Occurrence of Mating and Combat Behavior of the Rattlesnake Crotalus v. viridis. Journal of Herpetology, 1993. 27(4): p. 481-484.
71. Almeida-Santos, S.M. and O.A.V. Marques, Male-male ritual combat in the colubrid snake Chironius bicarinatus from the Atlantic Forest, southeastern Brazil.
72. Gans, C., J.C. Gillingham, and D.L. Clark, Courtship, Mating and Male Combat in Tuatara, Sphenodon punctatus. Journal of Herpetology, 1984. 18(2): p. 194-197.
73. Shine, R., et al., Ecology of cobras from southern Africa. Journal of Zoology, 2007. 272(2): p. 183-193.
74. Marshall, N.J. and R.L. Natasha, No Evidence of Female Choice for a Condition-Dependent Trait in the Agamid Lizard, Ctenophorus ornatus. Behaviour, 2001. 138(8): p. 965-980.
75. Tokarz, R.R., Mate Choice in Lizards: A Review. Herpetological Monographs, 1995. 9: p. 17-40.
76. Rhen, T. and D. Crews, Organization and Activation of Sexual and Agonistic Behavior in the Leopard Gecko, Eublepharis macularius. Neuroendocrinology, 2000. 71(4): p. 252-261.
77. Flores, D., A. Tousignant, and D. Crews, Incubation temperature affects the behavior of adult leopard geckos (Eublepharis macularius). Physiology & Behavior, 1994. 55(6): p. 1067-1072.
78. Bohme, W. and H. Nickel, Who was the first to observe parental care in crocodilesHERPETOLOGICAL BULLETIN, 2000(74): p. 16-18.
79. Vergne, A.l.L., et al., Parent-offspring communication in the Nile crocodile Crocodylus niloticus: do newborns’ calls show an individual signatureDie Naturwissenschaften, 2007. 94(1): p. 49-54.
80. Herzog, H.A., Jr., An Observation of Nest Opening by an American Alligator Alligator mississippiensis. Herpetologica, 1975. 31(4): p. 446-447.
81. Shine, R., et al., The Influence of Nest Temperatures and Maternal Brooding on Hatchling Phenotypes in Water Pythons. Ecology, 1997. 78(6): p. 1713-1721.
82. Lee, D.S., Possible Communication between Eggs of the American Alligator. Herpetologica, 1968. 24(1): p. 88-88.
83. Stahlschmidt, Z. and D.F. Denardo, Parental behavior in pythons is responsive to both the hydric and thermal dynamics of the nest. The Journal Of Experimental Biology. 213(Pt 10): p. 1691-1696.
84. Stahlschmidt, Z.R. and D.F. Denardo, Obligate costs of parental care to offspring: egg brooding-induced hypoxia creates smaller, slower and weaker python offspring. Biological Journal of the Linnean Society, 2009. 98(2): p. 414-421.
85. Bell, G., The Costs of Reproduction and Their Consequences. American Naturalist, 1980. 116(1): p. 45-76.
86. Niewiarowski, P.H. and A.E. Dunham, Effects of Mortality Risk and Growth on a Model of Reproductive Effort: Why the Shine and Schwarzkopf Model is Not General. Evolution, 1998. 52(4): p. 1236-1241.
87. Shine, R., “Costs” of Reproduction in Reptiles. Oecologia, 1980. 46(1): p. 92-100.
88.Shine, R. and L. Schwarzkopf, The Evolution of Reproductive Effort in Lizards and Snakes. Evolution, 1992. 46(1): p. 62-75.
89. Niewiarowski, P.H. and A.E. Dunham, The Evolution of Reproductive Effort in Squamate Reptiles: Costs, Trade- Offs, and Assumptions Reconsidered. Evolution, 1994. 48(1): p. 137-145.
90.Allan, J.L., Manipulation of Egg Production Reveals Costs of Reproduction in the Tree Lizard (Urosaurus ornatus). Oecologia, 1994. 100(3): p. 243-249.
91. Tinkle, D.W., The Concept of Reproductive Effort and Its Relation to the Evolution of Life Histories of Lizards. American Naturalist, 1969. 103(933): p. 501-516.
92.Wallace, B.P., et al., Egg components, egg size, and hatchling size in leatherback turtles. Comparative Biochemistry and Physiology, Part A, 2006. 145(4): p. 524-532.
93. Woodward, D.E. and J.D. Murray, On the Effect of Temperature-Dependent Sex Determination on Sex Ratio and Survivorship in Crocodilians. Proceedings B: Biological Sciences, 1993. 252(1334): p. 149.
94.Ford, N.B. and R.A. Seigel, An Experimental Test of the Fractional Egg Size Hypothesis.
95. Zhang, F., et al., Climate warming and reproduction in Chinese alligators. Animal Conservation, 2009. 12(2): p. 128-137.
96. Olsson, M., et al., Paternal Genotype Influences Incubation Period, Offspring Size, and Offspring Shape in an Oviparous Reptile. Evolution, 1996. 50(3): p. 1328-1333.
97. Shine, R., Reproduction in Australian elapid snakes II. Female reproductive cycles. Australian Journal of Zoology, 1977. 25(4): p. 655.
98. Shine, R. and P. Harlow, Maternal Thermoregulation Influences Offspring Viability in a Viviparous Lizard. Oecologia, 1993. 96(1): p. 122-127.
99. Burger, J., Incubation Temperature Has Long-Term Effects on Behaviour of Young Pine Snakes (Pituophis melanoleucus). Behavioral Ecology and Sociobiology, 1989. 24(4): p. 201-207.
100. Abell, A.J., Variation in Clutch Size and Offspring Size Relative to Environmental Conditions in the Lizard Sceloporus virgatus. Journal of Herpetology, 1999. 33(2): p. 173-180.
101. Congdon, J.D. and J.W. Gibbons, Egg Components and Reproductive Characteristics of Turtles: Relationships to Body Size. Herpetologica, 1985. 41(2): p. 194-205.
102. Ford, N.B. and R.A. Seigel, Relationships among Body Size, Clutch Size, and Egg Size in Three Species of Oviparous Snakes. Herpetologica, 1989. 45(1): p. 75-83.
103. Shine, R., A New Hypothesis for the Evolution of Viviparity in Reptiles. American Naturalist, 1995. 145(5): p. 809-823.
104. Denis, C.D. and W.J.F. Mark, The Mechanism of Temperature Dependent Sex Determination in Crocodilians: A Hypothesis. American Zoologist, 1989. 29(3): p. 973-985.
105. Newbold, T.A.S. and J.A. MacMahon, Consequences of Cattle Introduction in a Shrubsteppe Ecosystem: Indirect Effects on Desert Horned Lizards (Phrynosoma Platyrhinos).
106. Hellgren, E.C., et al., The Effects of Winter Burning and Grazing on Resources and Survival of Texas Horned Lizards in a Thornscrub Ecosystem. Journal of Wildlife Management. 74(2): p. 300-309.
107. Cardozo, G. and M. Chiaraviglio, Landscape changes influence the reproductive behaviour of a key ‘capital breeder’ snake (Boa constrictor occidentalis) in the Gran Chaco region, Argentina. Biological Conservation, 2008. 141(12): p. 3050-3058
Cite this Page
Diversities in reproductive modes and strategies amongst various reptile species. (2019, Mar 15). Retrieved from https://phdessay.com/diversities-in-reproductive-modes-and-strategies-amongst-various-reptile-species/
Run a free check or have your essay done for you
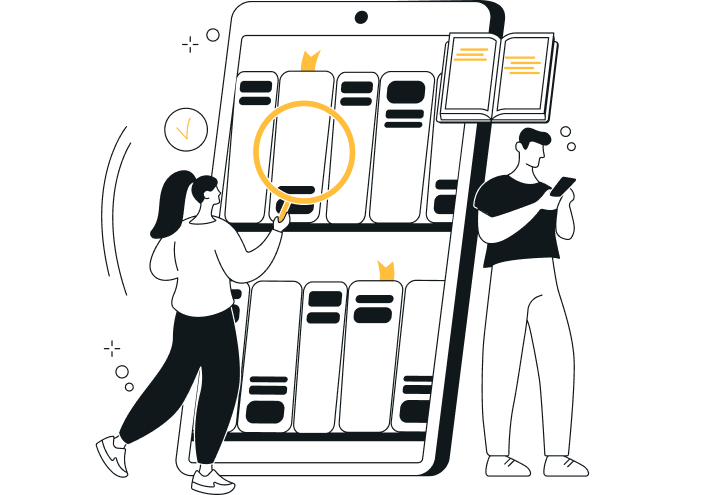