Smart materials
The primary characteristic of a "smart material" is that it has the ability to respond to external stimuli in a technically useful and technically controlled way. The words "technically useful" and "technically controlled" are emphasized since all materials respond to external stimuli of some sort or other (as a simple example, all materials respond to temperature by changing their volume), however, to be considered a "smart material" the response must be one that is useful in an engineering application.
Thus, any discussion of smart materials must include a consideration of the application of these materials. Animals and plants could be considered as consisting of a large number of smart materials, however, the scope of this article will be restricted to inorganic and organic materials that are used in a more traditional engineering sense. ) The term smart material often also has a historical context, only being applied to relatively new materials. For example, consider the simple bimetallic strip.
Bimetallic strips have been around for centuries and consist of two metals Joined so that the difference in the coefficient of thermal expansion causes the strip to bend in response to a change in temperature. This can be used, eg, to open or close a echanical valve or electrical circuit. The stimuli may either be provided by the natural environment or engineered into a structure that the material is part of. However, bimetallic strips are often not thought of as smart materials because they have been around and used for a long time.
Order custom essay Smart materials with free plagiarism report
Smart materials are also often characterized by the fact that they transform energy from one mode to another, eg, from electrical energy to mechanical energy. Smart materials are also often incorporated in so-called Smart Structures, which are structures that, as well as being the structural support of a building or vehicle, also have a further function. For example, a load-bearing structure that also measures the load that it is carrying is an example of a smart structure.
1. Classification schemes
How a material is considered or classified depends to some extent on the scientific or technical discipline that is considering the material. For example, a material scientist is interested in the internal structure and how this may change under the influence of an external stimulus, whereas an application engineer may be more concerned with the function of the material and what it can be used for.
Classification according to function
Smart materials can be classified according to the intended primary function, eg, as a sensor, actuator, or energy generator.
Piezoelectric material is an example of a class of smart materials that can be used in all three of these functions. When subjected to stress these materials generate an electrical voltage that can be used as a response signal and also to generate power. Alternatively, when subjected to a voltage these materials generate a mechanical stress. The interchangeable nature of stimulus and response is a common, but not universal, characteristic ot 1 Kirk-Othmer Encyclopedia of Chemical Technology. Copyright John Wiley & Sons, Inc. All rights reserved. vol. O smart materials. Materials that exhibit this interchangeable nature are often good candidates for smart structures with minimal external support requirements: one smart material both sensing and generating power and also capable of providing a mechanical or other response. Active Sensing Materials. The primary action of these materials is to transform one form of energy to another form of energy for, in most cases, a further device or material used to then generate a response. Active sensing materials may often be used to generate energy.
For example, the voltage developed by electric polarization of a material can be used to accumulate charge, and the solar cell both detects the presence of incident light and generates a significant voltage and current. Passive Sensing Materials. These materials have a passive response to external stimuli, ie, do not transform an energy source to another energy. Thus these devices need an external source of power to operate. They are not really "smart", but are included here for completeness since they do appear as sensors in larger smart systems.
An example of a passive response is a change in electrical conductivity due o, eg, a change in pH of a solution. Actuating Materials. The primary function is to transform one form of energy to another form as a response to some external stimuli and to perform an action.
Classification according to smartness
Another possible classification is according to the degree of independence of operation that the smart material needs to operate in a smart structure. In this case, materials that require the least external support (eg, external power or processing capability) being considered smarter.
A common example of a totally independent smart material is that used in photochromic sunglasses. In this case, the energy required to drive the response, darkening of the sunglasses is provided directly by the stimulus, the incident light.
Classification according to material properties
A further way to look at smart materials is to consider the primary physical-chemical property of the material that is used in smart applications. This is particularly useful in studying and understanding the operation of smart materials.
Crystal Structure Modification. A number of classes of smart materials have crystal structural properties that can be used directly for smart actuating or sensing applications. These include materials that undergo a crystallographic phase change (eg, shape memory alloys), materials that have an electrical polarization natural to the crystal structure that responds to external stress (eg, piezoelectric materials) and materials that undergo realignment ot internal domains, such as electro- and magnetostrictive materials.
Energy Absorption-Emission. This includes materials that absorb incident energy by an internal excitation process (eg, promotion of electrons, on absorption of a photon, to a higher energy band). These often have a de-excitation process that involves the emission of energy. Examples include semiconductor devices as light sensors and energy harvesting devices. Macroscopic Ordering. Includes (generally) mixtures of materials where the large scale ordering of the particles in the mixture induces a change in properties of the mixture.
Examples include electro- and magnetorheological fluids, 3 where an applied field induces particles in the fluid to align resulting in a change in viscosity. The Liquid Crystal Display can also be considered in this category. Chemical Interaction. Includes materials with sensitized surfaces that interact with target chemical or biological species. Examples include conducting polymers with functional groups that bind to chemical species and change the resistance of the polymer, and antigen coatings on microbalance mass detectors. Indicator chemicals that change color with, eg, pH, are another form of chemical sensor.
2. Smart materials overview
The following sections consider a number of these materials in more detail. Some sections deal with a particular type of smart material, whereas others deal with a class of material types. Inevitably, there are verlaps between these two, however, consideration of smart materials in this way is beneficial since, as discussed above, the view of the subject is colored by the point of view of the discussion.
Smart materials include such a wide range of materials and possibilities it is worthwhile viewing the subject from a number of angles. The following is not an exhaustive list of smart materials, but serves as examples of the more common smart materials. Many of the materials in Table 1 can be described as "responsive" rather than truly smart, ie, these materials can form one part of a smart system, either the sensor or ctuator, but require another responsive material for the actuator or sensor, respectively, to make up a complete smart system.
Only a few materials, eg, the photochromic sunglasses mentioned in the section classification schemes, are both sensor and actuator. Even materials that can be used as an actuator and sensor often cannot be engineered to perform both functions effectively at the same time. Thus, most smart materials are used in combinations to form smart systems. An example of a possible system is an antivibration device that uses a piezoelectric sensor to detect the vibration and a magnetorheological fluid as the damper.
The reterence section includes a number ot Internet web sites tor turtner practical information and as examples of the use and current availability of some smart materials. A number of these references are to commercial web sites as useful sites regarding the application and maturity of some of the technologies, but this in no way is an implied endorsement of the particular companies.
3. Piezoelectric and related
Phenomena Piezoelectric materials are materials that exhibit a linear relationship between electric and mechanical variables.
The electric polarization is proportional to the mechanical stress. Only materials with an electrically poled, anisotropic crystal stucture can form piezoelectrics; 'e, there must be an intrinsic electric field maintained in a particular direction throughout the material.
Thus the material must be pyroelectric. A feature of a pyroelectric material is the disappearance of this pontaneous electric field above the Curie point temperature. Piezoelectric devices are formed by raising the material above the Curie point temperature and then cooling in the presence of a strong electric field. The common term for this action is to say that the material is "poled" in the presence of the electric field. This results in partial or complete alignment of the spontaneous electric field within the material. A related material property is the ferroelectric effect.
All ferroelectric materials are necessarily both pyroelectric and piezoelectric. The ferroelectric effect is the electric olarization brought about by the complete or partial realignment of the spontaneous polarization resulting in a static electric field at the surfaces of the materials. (But note, a net field is not normally detected because the surface charge is rapidly neutralized by ambient charged particles. ) There are two principal types of materials that can function as piezoelectrics: the ceramics and polymers.
- Ceramics: The most widely used materials are the piezoceramics based on the lead zirconate titanate (PZT) formations, mixed sodium and potassium niobates, lithium niobate, and quartz. The advantages of these piezoceramics are that they have a high piezoelectric activity and they can be fabricated in many different shapes.
- Polymers: Poly(vinylidene fluoride), PVDF, is the most widely used polymer piezoelectric material. It has the advantages over ceramic devices of flexibility, formability and can be simply cut to shape. Polymer piezoelectric materials have lower authority (force and displacement) than ceramic devices.
The PVDF structure is a good example of the spontaneous electric field seen in these types of material. Composite structures consisting of a length of piezoelectric bonded to a nonpiezoelectric substrate can be used to convert the lateral change in dimension of the piezoelectric to a bending force. In this way, larger actuation strokes can be achieved. Piezoelectric materials are used in many different types of sensing and actuating devices and also for power harvesting.
Examples include Sensors: vibration, sound, accelerometers, pressure, ultrasonics, strain, power generation. Actuators: print heads, vibration suppression, speakers and buzzers, spark generators, ultrasonic ransducers, micropositioning and translation. Electrical components: filters and resonators.
4. Shape memory alloys and polymers
Metal shape memory alloys (SMAs) exhibit the properties of pseudo-elasticity and the shape memory effect. These alloys undergo thermomechanical changes passing from a martensitic phase at low temperature to an austenitic phase at higher temperature.
Shape memory effect
In the martensite phase, the alloy is softer and easily manipulated through large strains with a little change in stress, ie, it can be easily deformed. As the temperature of the alloy is increased above the critical transformation) temperature, it changes into the austentic phase. In the austentic phase, the alloy regains its high strength and high modulus and also reverts back to its original shape. Thus a SMA can be formed into shape above the transformation temperature, cooled below the transformation temperature, and formed into different shape.
Pseudo-elasticity
This occurs when the alloy is completely composed of austenite ('e, the temperature is above the transformation temperature). If the temperature is kept constant and the material is loaded, then at some point there will be a transformation to the martensite phase simply due to loading. The load is absorbed by deformation of the softer martensite phase, but upon unloading the martensite starts to transform back into austenite and the materials springs back into its original shape. Shape memory alloys (SMAs) can be divided into three functional groups: one-way SMAs, two-way SMAs, and magnetically controlled SMAs. The magnetically controlled SMAS show great potential as actuator materials for smart tructures because they could provide rapid strokes with large amplitudes under precise control. The most extensively used conventional shape memory alloys are the nickel-titanium, copper-zinc-aluminium, and copper- aluminium-nickel alloys. Due to their low cost, iron-based shape memory alloys are becoming more popular in smart structure applications.
Iron-manganese- silicon steels alloyed with chromium, nickel, and cobalt, and iron-manganese- silicon steels alloyed with nitrogen all fit into this category. As previously mentioned, the nickel-titanium alloys have been the most widely used shape memory alloys. This family of nickel-titanium alloys is known as Nitinol (Nickel Titanium Naval Ordnance Laboratory in honor of the place where this material behavior was first observed). Nitinol can be used in robotics actuators and micromanipulators that simulate human muscle motion.
The ability of Nitinol to exert a smooth, controlled force when activated is an advantage of this material family. SMAS have been used for military, medical, safety, and robotics applications. Specific usages include hydraulic lines, medical tweezers, anchors for attaching tendons to bones, eyeglass frames, control of hot house windows, underwire brassieres, and ntiscalding valves used in water taps, and shower heads.
Shape memory polymers.
Shape memory polymers (SMP) are polymers (polyurethane based thermoplastics) that can be heated (above the glass transition temperature), deformed, and cooled to retain the deformed 6 shape.
Upon heating above the transition temperature, the material softens and returns to the shape that it had prior to deformation. Advantages of SMPs over metallic SMAS include light weight, high recoverable strains (up to 400%), injection moulding (to form complex shapes), low cost and SMPs have shape recovery temperatures selectable between A30 and 708C. The SMAs, however, have superior force characteristics and can operate at higher temperatures.
5. Electrostrictive materials
Electrostrictive materials are materials that exhibit a quadratic relationship between mechanical stress and an applied electric polarization (Fig. ). Electrostriction can occur in any material. Whenever an electric field is applied, the induced charges in the material attract each other resulting in a compressive force. This attraction is independent of the sign of the electric field. The strain in the material lies along the axis of the induced polarization, which is preferably the direction of the applied lectric field. Electrostriction is a small effect and, in contrast to piezoelectric materials, electrostrictive materials show a large effect near the Curie temperature, especially for ferroelectric substances, such as members of the perovskite family.
Typical electrostrictive materials include such compounds as lead manganese niobate:lead titanate (PMN:PT) and lead lanthanium zirconate titanate (PLZT). Electrostriction is used in actuators for accurate and fine positioning. Electrostrictive translators are less stable than piezoelectric devices with greater sensitivity to temperature. The one advantage they offer is lower hysteresis than piezoelectric materials at temperatures typically >1
6. Magnetostrictive materials
The same type of material response as that seen in electrostrictive materials discussed above can be observed when the stimulus is a magnetic field.
Shape changes are the largest in ferromagnetic and ferrimagnetic solids. The repositioning of domain walls that occur when these solids are placed in magnetic field leads to hysteresis between magnetization and an applied magnetic field. All of these effects disappear when the ferromagnetic material is heated above its Curie temperature. Ferrimagnetic materials have macroscopic properties similar to ferromagnetics; however, their microscopic properties are different. The magnetic dipoles of a ferromagnetic solid are aligned parallel to each other; whereas in a ferrimagnetic the alignment can be either parallel or in other directions.
Materials that have shown a response to a magnetic stimuli are primarily inorganic: alloys of iron, nickel, and cobalt doped with rare earths.
TERFENOLD, an alloy of terbium, dysprosium, and iron, with x between 0. 27 and 0. 30 and y between 1. 90 and 1. 95, is the most effective magnetostrictive material and has been escribed as the "200 lb" gorilla of magnetostrictive materials. The name TERFENOL is an acronym for two of the elements present in the alloy and NOL refers to the Naval Ordinance Laboratory where this type 7 of material behavior was developed.
Magnetostriction occurs at its fullest potential in crystalline materials. Cost still appears to be one of the hindrances to magnetostrictive materials becoming commercially important. Piezoelectric materials are generally more compact and require less energy to operate than magnetostrictive materials. Magnetostrictive materials ('e, TERFENOL-D) are useful where high force, igh power, and a long stroke are required or where the high drive voltages typical of the piezoelectric materials cannot be tolerated.
7. Electro- and magnetorheological materials
Electrorheological and magnetorheological materials are fluids that exhibit a dramatic change in viscosity with an applied electric or magnetic field. The fluid can change from a thick oily consistency to virtually a solid substance within a millisecond. There are a wide assortment of electrorheological and magnetorheological fluids, which are usually a uniform dispersion or suspension of particles within a fluid. A typical example of an electrorheological fluid is a mixture of cornstarch in a silicone oil. The mechanism of how electrorheological fluids work is simple.
When there is no electric field the particles in the fluid are distributed randomly and are free to move in the fluid. In an applied electric field the particles orient themselves in ?ber-like structures (fibrils) that are much harder to move and impede the flow of the fluid, dramatically increasing the viscosity of the fluid-particle mixture. Typical magnetorheological fluids consist of tiny iron particles uspended in oil and have a similar behavior to the electrorheological fluids except initiated by the application of a magnetic field.
Electrorheological materials
Electrorheological fluids are nonNewtonian fluids, 'e, the relationship between shear stress and strain rate is nonlinear. The changes in viscous properties of electrorheological fluids are only obtained at relatively high electric fields, in the order of 1 kV/mm. The practical applications of electrorheological fluids center around their abilities to transfer shear stresses and of acting as a variable damping material in an electric field. They have been demonstrated in shock absorbers, brake systems, clutches, vibration damping, control valves, and actuators.
An illustrative application of electrorheological fluids is their use as a smart space material. In this application, a single-link flexible-beam was constructed in a sandwich confguration with ER fluids distributed along its length. When the beam is to be rapidly moved back and forth, the ER fluid is not energized, providing flexibility during the transient response period of the maneuver (for speed). At the end point of the maneuver the beam is made rigid (for stability).
Magnetorheological materials
Magnetorheological fluids are the magnetic equivalent of electrorheological fluids. An advantage over the ER fluids is that high voltages are not required to actuate the MR fluids. These fluids are under development for use in shock absorbers, vibration damping, exercise equipment and surface polishing of machine
8. Photoresponsive and sensitive materials
There are several different types of material families that exhibit different types of light transmission-absorption or other responses to a stimulus.
These include lectrochromism (a change in color as a function of an electrical field); thermochromism (color change with heat); photochromic materials (reversible lightsensitive materials); photographic materials (irreversible light-sensitive materials); photostrictive materials (shape changes due to light usually caused by changes in electronic structure); fluorescence (emission of light (photons) at a different wavelength to the incident light).
An interesting material with both electro- and thermochromism behavior, V02, was evaluated for a smart window application. Materials are being developed to exhibit both photochromic and photographic ehaviors and one such system is based on a substituted indolinospirobenzopyrene embedded in a polystyrene matrix. This system acts as a photochromic system at low exposure in the ultraviolet (uv) range and at high exposure it functions as a photographic system. The image can be devisualized by heat and can be restored many times witn uv irradiation.
Another interesting application is the use ot polymers that fluoresce or change color in the presence of particular metal ions. This is being tested as a corrosion sensor with optical fibers coated with an appropriate polymer r with the polymer incorporated in a composite structure. The system is optically excited and the presence of light at the fluorescence wavelength indicates metal ions (and thus corrosion). Thermochromic materials find use in security devices and in thermal mapping applications.
All semiconductor materials exhibit an electrical response to incident light due to the electron absorption of photons, of sufficient energy, and promotion of the electrons to the conduction band (change in resistance) and across doping gradients, as in diodes (generation of current and voltage) in solar cells. Of particular interest in this rea is the development of non-silicon systems, which may results in cheap, flexible, robust, and easy to transport and deploy solar energy collectors.
9. Chemical and biochemically sensitive materials
The most widely known classes of chemically sensitive materials are the pHsensitive materials that include the acids, bases, and indicators. The most interesting of these for smart applications are the indicators. These materials change colors as a function of pH and are usually totally reversible. Indicators have also been used in the development of novel chemical indicating systems.
Devices based on the ermeability of organic vapors through polymeric films or porous polymeric plugs, and subsequent reaction with an indicator, are used for monitoring the condition of time and/or temperature sensitive items, eg, pharmaceutics, foods and other perishables. The system is activated by crushing the vial releasing the volatile component, which then slowly permeates through the film reacting with the indicator to give a visible color change. This change is 9 dependent on both time and temperature.
Other examples of pH-sensitive materials include the smart hydrogels and smart polymers (see below in EAPs). There is increasing interest in the detection of other chemicals, particularly in the detection of chemical warfare agents, environmentally noxious chemicals, and the control and monitoring of (engine) emissions. Semiconductor film sensors based on metal oxides (eg, Sn02, ZnO, Ti02, W03) are used to measure the concentration of toxic and flammable gases.
These devices operate at several hundreds of degrees and a chemical reaction between the gas and the metal oxide changes the electrical conductivity of the oxide. The conductivity is a function of the temperature and gas concentration. Higher temperature devices (to $10008C) have been demonstrated using Sic and SrTi03 systems. At low temperatures, the gases interact with the metal oxide by a chemisorption mechanism. The chemically adsorbed particles receive a partial charge and the opposite charge is made available to the oxide as a tree electron to increase its conductivity.
Metal oxide sensors (MOS) are finding applications in the automotive industry monitoring the composition of exhaust gases. Many actions in organic systems are governed by highly selective reactions that are in effect molecular recognition mechanisms. The molecules responsible for these rocesses are highly selective in the molecules that they bind to ('e, recognize) and can be used in engineered devices as sensors. An example of this is coating piezoelectric material with a selective material and then detecting the increase in mass of the coating as the target molecule binds to the coating.
Being organic in nature means that a wide range of ways to modify these molecules are available, eg, light stimulated regions that change their fluorescence frequency in the presence of the target molecule. Conducting polymers can also be tailored to respond (by a change in resistance) to ifferent chemicals. In this case, the response is rather broad (ie, not very selective), however, systems have been developed with a number of polymers treated to react to different types of chemicals to obtain "fingerprints" that are specific to particular chemicals.
These systems generally have a limited lifetime and increasing the life and stability of the conducting polymers is the main challenge facing their acceptance as sensors. A novel proposal for chemical detection is the use of molecularly imprinted plastics (MIPs). In this case, target molecules are imbedded in a (porous) plastic matrix that is pplied to some sensing-transducer device. The target molecule is then dissolved away leaving a physical imprint in the plastic matrix.
On exposure to the molecule in a gas or liquid the physical imprints left in the plastic are selective for the target molecule and collect it, altering the properties (eg, mass) of the plastic.
10. Electroactive polymers (actuators)
An electroactive polymer (EAP) is a polymer that exhibits a response to an applied electrical stimulation and often also develops an electrical response to a mechanical stimulation. Some of these materials have been touched on in previous sections.
Cite this Page
Smart materials. (2018, Jul 01). Retrieved from https://phdessay.com/smart-materials/
Run a free check or have your essay done for you
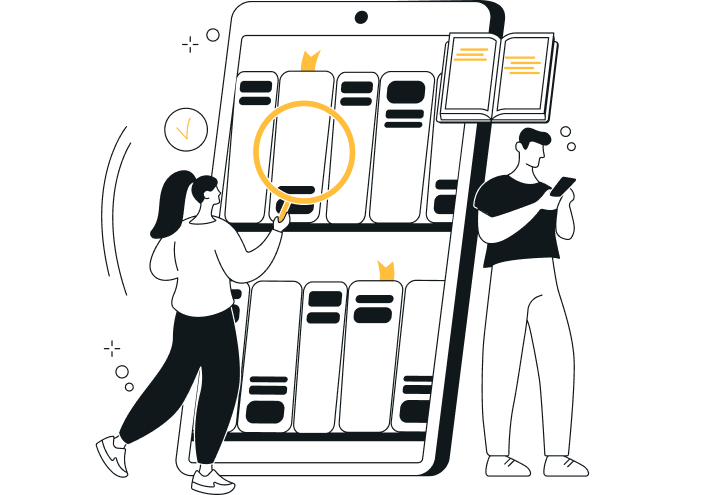